Citation: Nogueira J, Borghi T, Vergani M, “Choosing the Right Connectivity for Drug Delivery and Medical Products”. ONdrugDelivery Magazine, Issue 103 (Dec 2019), pp 11-15.
Juan Nogueira; Tommaso Borghi and Marco Vergani discuss the factors that need to be taken into account in the selection of connectivity technology for a drug delivery device or other medical product.
In the world of drug delivery – and other monitoring and personal medical products – there are “before and after iPhone” eras. Such medical devices used to have limited capabilities to interact with the user – such as basic LCD displays and push buttons, small memory to store the collected data, unfriendly user interfaces and no link or communication between the device and the user. The user had to rely on paper-based log sheets to note the device readings (e.g. glucose levels) and decide what action to take on their own (e.g. insulin injections). Using these error- prone and punctuated data recording methods, it was difficult to find patterns, and doctors had to spend added time with patients to review sparse (e.g. lack of measurement time information) and incomplete data.
“The number and feature sets of connectivity technologies have grown to such a level that the selection of the most appropriate one is a critical decision that will define the connectivity function and the product user experience.”
The introduction of the iPhone and subsequent spread of smartphones as the personal data and communication device – in parallel with the maturity of new low-power and inexpensive short-range communication technologies – has changed that landscape completely. The smartphone was not only able to provide a new colourful and interactive user interface and a communication link between the device and the user, it could also store many device data measurements sets, with the corresponding time stamps (i.e. complete data sets), and share that data with the doctor. Furthermore, that data collection could be uploaded to a database to be analysed with computer-assisted algorithms to extract data patterns and sequence relationships.
In this sense, smartphones have been used as a communication gateway between the medical device and the data backend. Bluetooth has been the dominant technology to enable connection between the smartphone and the personal medical device, and cellular communication for the link to the database. Today, these databases have evolved to become the cloud, where data is not only stored but also processed and analysed using advanced and complex algorithms.
This allows us to move all or part of the data processing to the cloud but has a resultant impact on the required data link capacity. The more that processing is done in the cloud versus the device, the higher the requirements for that data communications link. This trade-off can today be well balanced as small micro-controllers that are part of every Internet of Things (IoT) device can run algorithms that would formerly have needed high computer processing power.
The number and feature sets of connectivity technologies have grown to such a level that the selection of the most appropriate one is a critical decision that will define the connectivity function and the product user experience. Some of these new technologies, including low-power wide-area networks (LPWANs), will enable the elimination of the smartphone interface. Further to that, the future availability of 5G and its advanced wireless technologies that provide low latency and ultra-high reliability will enable new levels of personal medical devices not viable today.
CONNECTIVITY TECHNOLOGIES LANDSCAPE
The various connectivity technologies can be classified using different performance parameters that can be inherent to the technology itself – like range, data rate or power consumption. Or they can be classified by implementation attributes like cost, size, etc. Table 1 summarises these connectivity options with some examples of typical medical use cases.
Technology | Use Case | Cost | Range | Bit rate | Power | Comments |
NFC/RFID | Medical adherence | Low (tag) High (receiver) |
cm | 100s of kbps | 0 (tags) 100s mA (receiver) |
Requires direct patient involvement to initiate the communication. Needs app/smartphone to connect with the cloud. |
BLE | Medical adherence, wearables | Low | m | 100s of kbps | 5-10 mA | Needs app/smartphone to connect with the cloud |
Wi-Fi | Home monitoring, telemedicine, hospital equipment |
Low | 10s of m | 10s of Mbps | 10s mA | Needs modem/router to connect with the cloud |
Zigbee | Home monitoring, telemedicine, hospital equipment |
Low | Up to 10s of m or more in meshed networks | 100s of kbps | ~10 mA | Needs hub to connect with the cloud. Requires direct patient involvement to setup the ZigBee network. |
LPWAN – Unlicensed LoRa SigFox |
Elderly care, monitoring open/close events with no real-time requirements | Low | 10s of km | 100 bps to 10s Kbps | 10-30 mA | Direct access to cloud, no need for patient interaction to initiate the communication. Possible wide coverage by areas. |
LPWAN – Licensed NB IoT LTE-CatM |
Real-time applications, elderly care, emergency management, open/close events | Medium | 10s of km | 100 bps to 1 Mbps |
~200 mA | Direct access to cloud, no need for patient interaction to initiate the communication. Future global coverage expected |
5G | Real-time applications | High | 10s of km | 100 Mbs to 1 Gbps |
100s mA | Can provide bidirectional communication with ultra-low latency and high data rate. Enables applications with real- time user feedback |
Table 1: A summary of connectivity options with examples of medical use cases.
The value range of these parameters may also determine if one connectivity solution is suitable for a specific medical device. Figure 1 shows classification of various devices based on the range and latency parameters. But there are more parameters that need to be considered to make the most appropriate selection.
“Given that the requirements and constraints of different devices vary significantly, there is no one perfect solution.”
A very broad spectrum of devices, for instance, needs to be considered for the drug delivery market – ranging from drug monitoring devices (miniaturised devices often powered by primary coin cell batteries) to autoinjectors (bigger devices with motors and rechargeable batteries). Given that the requirements and constraints of different devices vary significantly, there is no one perfect solution. But understanding the trade-off relationship where the improvement of one parameter comes at the cost of another (i.e. range versus power consumption) allows confident and informed decision making.
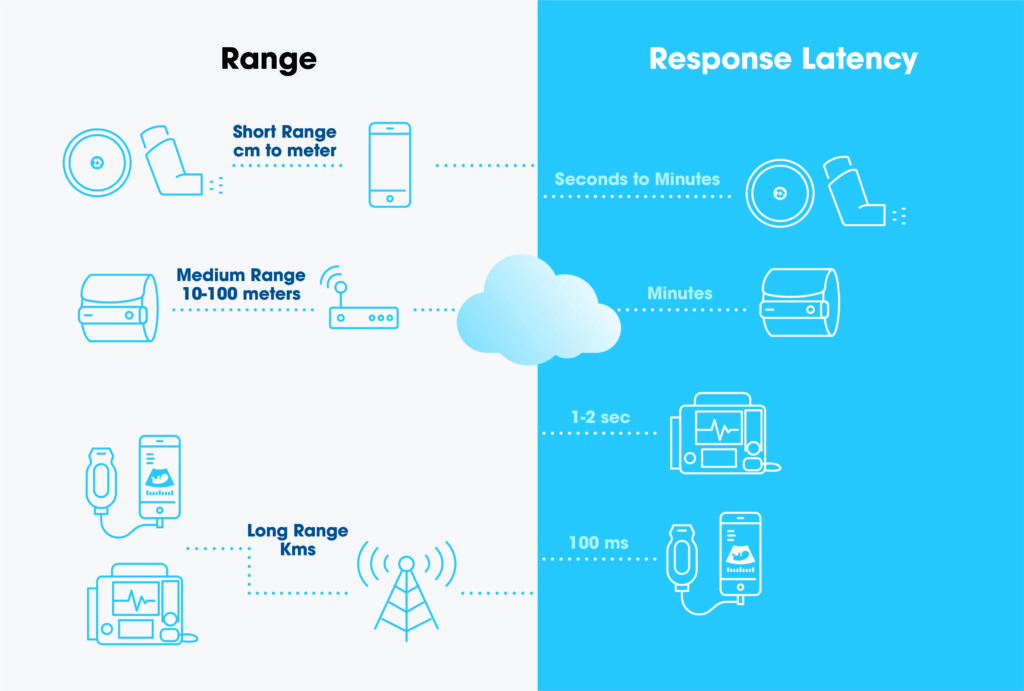
Figure 1: Example of device classifications based on range and latency.
In the following section we explore some questions that may help in selecting the most appropriate connectivity solution from several points of view – technical, user interface, cost, etc.
“The complexity of
setting up and managing the communications can be a decision point.”
HOW TO SELECT
The way the device is going to communicate and interact with the user (or patient) and caregiver, the device size, the available power, the amount of data to be transmitted, the market region in which the device is going to be used and the need for real-time feedback will each have a direct impact on the selection of connectivity technology. Also, the complexity of setting up and managing the communications can be a decision point – older patients, for example, may have difficulties or lack of knowledge in how to pair a device with a smartphone, or how to access Wi-Fi networks in different locations.
The following are some of the questions a drug delivery device maker can ask to help guide decision making:
Data reader
What type of reader is needed? Is the end user’s smartphone part of the ecosystem?
The early days of connected medical devices were dominated by custom protocols and readers. Part of the reason was the requirement for highly reliable channels that were not available on smartphones at that time – but also the complexity of the validation process of a non-medical device in the loop. But the market is adapting very quickly to the latest trends and, along with it, there is a more open attitude from regulators.
Several players have adopted this approach and created smartphone-based apps as the solution to interact with their devices. In this case, the smartphone will be used as a simple gateway that moves data from the device to the cloud by using a short-range communication technology like Bluetooth Low Energy (BLE) or near-field communication (NFC). But even this selection, which appears simple and straightforward, carries some caveats. For example, selecting NFC allows for a very low-power-consumption device profile and has very low cost but it would require user interaction to read the data, which will affect the user experience. Furthermore, NFC is not present in all smartphones.
Data
What type of data is generated and what type of data will be transmitted?
Every application has specific needs. For example, a wearable ECG monitor requires continuous and real-time streaming of data with a throughput of tens of kB/s, while an autoinjector only demands hundreds of bytes transmitted per day.
Power
What is the peak current that is required by the connectivity solution?
Small devices based on coin cells may not be properly driven at low temperature, where the battery cannot provide the peak pulses needed for some RF communication technologies. Cost and size of the device might increase due to extra storage or capacitors. Also, the required peak current may disqualify the use of long-range technologies with some coin cell operated devices. On the other hand, devices with rechargeable batteries and use cases where frequent recharge of the device is acceptable will enable the use of power-hungry technologies.
“A very common dilemma in the industry is whether to involve patients in the communication process between the device and the reader or make the transmission transparent and seamless to their eyes.”
RF performance
How fast will the energy stored in the battery be consumed? Will the connectivity functionality demand peaks of high current to transmit data? Is the device intended to be connected while in use? Are there batteries or metal shields in the proximity of the RF block?
It is recommended that a detailed power and energy budget analysis is performed, based on an accurate definition of the device use cases, and evaluated over the device operating temperature range. Consider maximum transmission power, lack of network coverage if applicable (cellular network-reconnect takes time and is a power-hungry process) and retransmissions as worst-case scenarios. Special attention must be applied in devices that are handled, gripped by a hand or carried in a pocket, near the body or where metals are nearby. The antenna can be detuned and the RF link performance heavily reduced. RF simulations are suggested, evaluating different antenna positions.
User experience
How is the device going to be used?
What is the patient’s role in communicating? When will data be transmitted?
A very common dilemma in the industry is whether to involve patients in the communication process between the device and the reader or make the transmission transparent and seamless to their eyes. There are pros and cons for each solution.
Maintenance
Is a firmware update over the air required?
Connectivity technologies with low-data-rate solutions may not be supporting this function. Case-by-case evaluation is needed. For safety purposes, it is recommended to keep the previous firmware image of the code available until the new one is fully validated – at the cost of space because a bigger flash memory is required for storage.
Connectivity costs
Does the price to the user include the cost of ongoing connectivity?
Does the cost of connectivity fit into the target total cost of the device? Will recurrent connectivity costs associated with the device be accepted? Cellular connections may have a monthly fee, whereas connectivity via BLE or Wi-Fi are no-cost options adding no repeated cost to the user.
Security
What is the level of security and data protection required?
Does the connectivity standard being used provide methods to guarantee the level of security, authentication and data protection required? Is this true for all targeted geographies?
Mechanics
What are the mechanical constraints when it comes to fitting connectivity into the existing device?
Smart connected devices are often the result of retrofitting electronics into devices that were not designed to include extra components like PCBs, batteries and antennae. Much time is often spent in the design of custom solutions to cope with the tight mechanical constraints set by the legacy product.
Product cost
What is the budget available to add connectivity to a medical device? Is an application-specific integrated circuit (ASIC) the way to go?
While connectivity is a clear trend in this market, there is still little literature around the added value of these devices in specific use cases. Pharma companies must still prove the added value of connectivity to drive price and reimbursement increases. For this reason, pressure remains high to minimise extra costs associated with these types of devices and significant effort is spent on aggressive cost optimisation, especially where the unit volumes can be very high – e.g. disposables (Figure 2). A BLE chip might represent a major share of the costed bill of material.
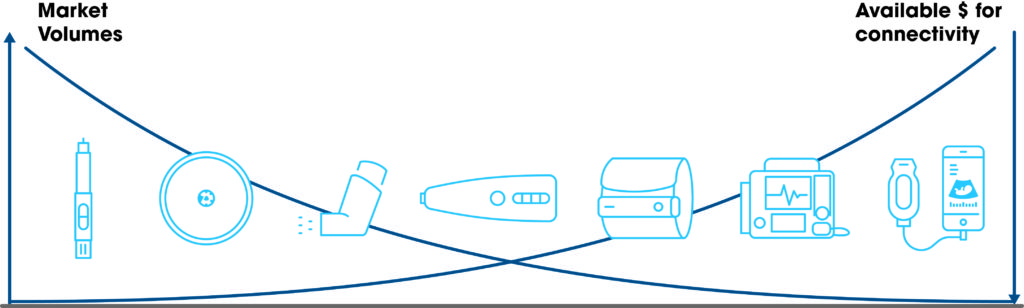
Figure 2: Budget available for connectivity versus the market volume.
Regulation
In what environment is the device meant to be used (hospital, home, indoor, outdoor)?
It is mandatory to consider all regulatory constraints and guidelines to design a properly connected device. The main driver is the quality of connection service that must be guaranteed in accordance with the risk profile of the application.
Latency
Does the device require real-time and low-latency communication with the cloud while it is being used?
5G technology is going to enable new handheld medical devices which are today limited to trained professionals because of the usage complexity. Some assistance and usage feedback must be provided in real time so that the user is able to take the measurements in a proper way. A handheld ultrasound device is an example of this type of device (Figure 1).
A CONSEQUENTIAL DECISION
The selection of connectivity technology can have a dramatic impact on the performance and usability of a device. The range of options continues to grow as new technologies are developed and mature. A clear and deep understanding of the technology performance and characteristics is an essential element in the successful design of a device that fulfils all aspects of its intended performance.
First and foremost, drug delivery and medical devices are intended to deliver therapeutic and clinical benefit. That benefit can be supported and further enhanced by the addition of connectivity technology. The right connectivity technology will support adoption and adherence by meeting the requirements of all the various stakeholders. The wrong connectivity technology can have the opposite effect. Selection is a consequential decision. Understanding the details of connectivity technology, the limitations and the trade offs is the best approach to de-risk the design, development and market adoption of a device.
Previous article
PRODUCT SHOWCASE: Injay – Connected Prefilled SyringeNext article
THE CURRENT STATE OF PLAY IN CONNECTED DEVICES