To Issue 140
Citation: Nazarzadeh E, Pritchard J, “Creating a Platform for Nebulisation of a Wide Range of Drug Types and Formulations”. ONdrugDelivery, Issue 140 (Nov 2022), pp 37–42.
Elijah Nazarzadeh and John Pritchard discuss the potential of the inhalation route for both treating respiratory disorders and systemic delivery, consider the limitations of current inhalation technologies and introduce Nebu~Flow – a novel class of nebuliser technology that represents a major step in the ability to deliver drugs efficiently to the lungs.
“Drug delivery via inhalation can be an alternative to the oral and parental drug delivery routes, with the advantages of bypassing gastrointestinal and hepatic metabolism and reducing the side effects.”
According to the WHO, respiratory diseases, such as asthma and chronic obstructive pulmonary disease (COPD), are the leading causes of death and disability in the world.1 The British Lung Foundation estimates that one in every five people in the UK will be affected by a lung disorder during their life, with staggering annual cost of £11 billion, while it is estimated that more than 200 million people suffer from COPD globally, from which about 3.2 million die each year.1–3 Furthermore, the covid-19 pandemic, which has claimed more than 4.5 million lives, mainly due to respiratory causes, has highlighted the importance of healthy lungs for fighting respiratory infections.3
These disorders are usually treated by the inhalation of aerosols, where the effective delivery of medication is crucially dependent upon the droplet size distribution and inhalation pattern.4–5 It is generally believed that droplets larger than 10 μm are caught in the nose and throat, while droplets larger than 5 μm can reach the upper airways. Only droplets smaller than 5 μm can reach the small bronchi and alveolar regions, where the chances of drug adsorption are higher.
In addition, there is growing interest in exploiting the large surface area of lungs for systemic drug delivery. It has been shown that drug delivery via inhalation can be an alternative to the oral and parental drug delivery routes, with the advantages of bypassing gastrointestinal and hepatic metabolism and reducing the side effects.6 The alveolar region of the lungs provides a large surface area (up to 75 m2) with a long residence time and a higher chance of uptake of the drug to the blood stream, making it an ideal target for systemic drug delivery.7 However, current devices have limited ability to generate aerosols smaller than 2 μm, which are necessary to target the alveolar region.
INHALATION DRUG DELIVERY DEVICES AND UNMET NEEDS
Current inhalation drug delivery devices are mainly categorised as either inhalers or nebulisers. Inhalers are small, portable devices that normally contain one month’s therapy, whereas nebulisers are relatively larger devices, dispersing liquid formulations from a unit dose package in the form of an aerosol.
Correct use of inhalers requires specific manipulation and breathing techniques, which results in a high degree of misuse amongst patients, especially for those of older ages.8–9 On the other hand, nebulisers can aerosolise and deliver medication during tidal breathing, providing an easier drug delivery method that does not require patients to be trained in a special inhalation technique.
Furthermore, nebuliser drug delivery provides an easier formulation step, where the drug dose can be easily adapted during the dose-ranging studies. This is of significant importance in the early stages of drug development, accelerating clinical trials and regulatory approvals. The use of nebulisers in clinical trials has increased in recent years and has played an important role in enabling new drugs for covid-19 treatment; 69 of the clinical trials started in 2020–21 for the treatment of SARS-CoV-2 used a nebuliser, compared with only 16 drugs trialled using an inhaler.10
Because of the significant variability in nebuliser performance between different makes of device, regulators treat a new drug-nebuliser formulation as a combination product, granting market authorisation for the drug to be administered only using that specific device. This means that drugs nearing the end of their patent life can be repurposed as nebulised treatments and still retain a degree of market protection. It should be noted that the market protection of such a combination product can be enhanced by the intellectual property protection of the nebuliser device. It has also been shown that the development and launch of a new drug in a nebulised form before that of an inhaler can have a significantly better return on investment.11
A search of clinical trials in 2020–21 showed that almost 54% of clinical trials used a nebuliser, compared with the historic average of 25%. Also, the market trend shows that the number of doses of drug delivered by nebulisers has increased by 6%, compared with only a 3% increase for the number of doses delivered by inhalers.11
However, despite advances in current nebuliser technologies, including jet and mesh systems, existing state-of-the-art devices still have some limitations, namely:
- Limitations on controlling the aerosol particle size
- Sub-optimal performance
- Poor user experience
- Limited range of acceptable formulation properties (e.g. viscosity, surface tension).
This article provides an overview of current nebuliser technologies on the market and their limitations. This is followed by an introduction to the Nebu~Flow® surface acoustic wave (SAW) nebulisation technology, which is the most recent development in the field, and some its advantages over current devices.
“Despite advances in current nebuliser technologies, including jet and mesh systems, existing state-of-the-art devices still have some limitations.”
Jet Nebulisers
Jet nebulisers have been in use since the 1850s and have the highest share of the market. They are developed based on the Venturi principle, using a high-pressure flow of air (or oxygen) through a nozzle to aerosolise liquids. At the Venturi, air pressure drops and the gas velocity accelerates. This generates a negative pressure that sucks the liquid from the reservoir to this point and breaks it into the form of droplets (Figure 1, left).
The generated aerosol has a range of large and small droplets, with the large ones captured by a baffle and recirculated into the reservoir and the smaller ones leaving the area for inhalation. The aerosol droplet size from a jet nebuliser varies highly with the design of the system, which results in a significant variability in jet nebulisers from different companies.10 The use of high-pressure air and multiple passes of the liquid through the Venturi makes this system unsuitable for a range of formulations. For example, formulations of fragile biologic drugs can be degraded through the multiple passes, while formulations with a surface-active molecule usually result in foaming in the chamber and cannot be nebulised efficiently.12
Jet nebulisers are usually inefficient and have a high residual drug volume that cannot be aerosolised (>0.5 mL).13 This, combined with the noise from the pump and cleaning procedures, makes them an unfavourable device for patients.
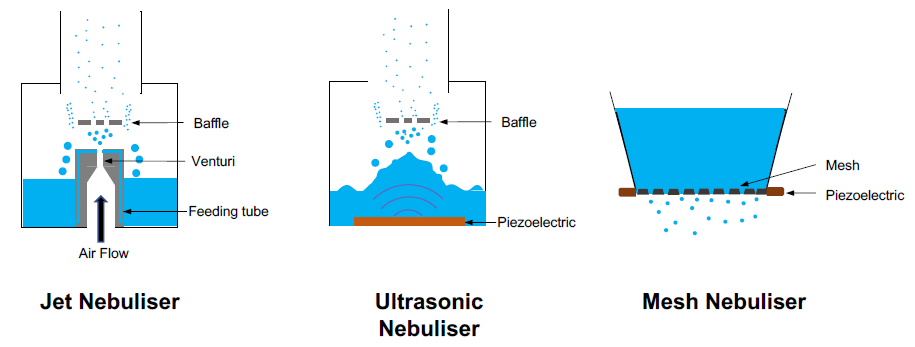
Figure 1: Schematics showing the working principles of current nebuliser technologies – jet nebuliser (left), ultrasonic nebuliser (middle) and mesh nebuliser (right).
Ultrasonic Nebulisers
The first use of ultrasonic nebulisers can be dated back to 1950s. They employ the energy from an ultrasonic beam to disperse liquids in form of aerosols. The wave of ultrasound is produced by rapid vibration of piezoelectric crystal, which transfers its energy to the liquid. The piezoelectric crystal is usually placed at the bottom of the liquid reservoir, forming a crest from which droplets are detached (Figure 1, middle). Like jet nebulisers, large droplets are captured by a baffle and recirculated within the liquid reservoir.
Ultrasonic nebulisers usually employ frequencies in the range of up to few megaHertz, which can generate cavitation and increase the temperature of the liquid significantly. The excessive heat can denature the API, especially with macromolecules. Additionally, suspension formulations usually settle at the bottom of the reservoir and cannot be dispersed as an aerosol. These technical challenges have resulted in limited use of ultrasonic nebulisers, which are mainly used for the nebulisation and delivery of saline solutions.
“Nebu~Flow technology has been used successfully to nebulise a range of placebo and commercially available formulation types with a wide range of physical properties, including those with very low surface tension and high viscosity.”
Mesh Nebulisers
Mesh nebulisers were first developed in the early 1990s. Early mesh nebulisers were based on a technology originally developed for ink-jet printing, where the liquid was actuated indirectly. In one of the first designs, piezoelectric crystals vibrate around a feeding tube, which forces liquid through a mesh (pore size of 5 μm), which then creates an aerosol. Later forms of mesh nebuliser, where the piezoelectric crystal vibrates the mesh directly, are known as vibrating mesh nebulisers (Figure 1, right).
In general, the orifice sizes of current mesh nebulisers are in the range of 2–5 μm. The small orifices of mesh nebulisers result in a number of inherent technical limitations. As these orifices are very small, they tend to get blocked easily and cannot disperse suspension formulations when the particle in suspension has the same size as the orifice. Also, formulations with low surface tension usually drip through the orifices and flood the system, resulting in inefficient nebulisation. Finally, the passage of fragile biologics through the small orifices, combined with their lengthy exposure to the vibration within the drug reservoir, can denature these macromolecules.
While mesh nebulisers have increased the overall use of nebulisers, their inherent limitations in the delivery of different formulations results in a limited availability of drugs for patients. Also, the main patents for mesh nebulisers are expiring, which may discourage their adoption in the development of new drug-nebuliser combinations.
Unmet Needs
Despite the ongoing developments of nebuliser technologies, there are still significant unmet needs. As discussed, not only may complex formulations lose potency, the output rate and droplet size of nebulisers depends in large part on the physical properties of the formulation, such as viscosity and surface tension.14 Furthermore, suspension formulations are unsuitable for ultrasonic nebulisers and may block the micron-sized pores in mesh nebulisers.14
In addition, the droplet size is a fundamental function of the design of a nebuliser and can be difficult to change, which can clearly impact the likely efficacy of the product by targeting the wrong location in the lungs.15 A tuneable nebuliser would offer significant advantage in optimising the efficacy and safety profiles of a new drug. Finally, there is still room to improve the patients’ burden of disease when it comes to factors such as cleaning and treatment time.16 Thus, there has been ongoing research to find novel ways to create droplets of a size and output rate suitable for application as a nebuliser.
NEBU~FLOW TECHNOLOGY
One of the leading contenders to overcome the challenges of current nebuliser technologies is surface acoustic wave (SAW) atomisation. The SAWs are generated by an interdigitated transducer on the surface of a piezoelectric crystal, creating a Rayleigh wave with a nanometer amplitude, which travels on the surface of the material. These SAWs can transfer their energy (in the form of mechanical energy and acoustic pressure) into the liquid on their propagation path,17 dispersing the liquid in the form of aerosols.
The use of SAWs as a means of nebulisation was first reported by Kurosawa et al in 1995, promising development of a pocket-size nebuliser.18 However, although SAW-based nebulisation has been the focus of many research activities,19,20 no commercial product has yet been developed. These studies have proven that the use of SAWs is a “soft” method for nebulisation of fragile biologics, such as plasmid DNA and siRNA, which retain their biological activity post-nebulisation.20,21 However, for one design of a SAW nebuliser, studies showed that only 7%–10% of the loaded API reached the lungs.22
The main challenge of nebuliser technologies is controlling the aerosol droplet size for efficient drug delivery.
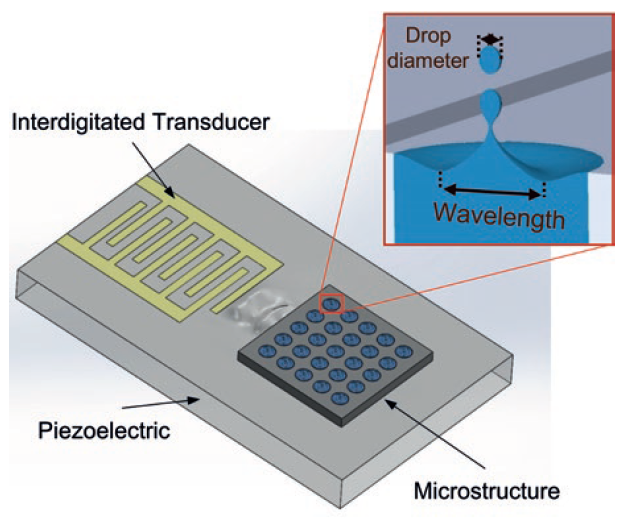
Figure 2: Schematics showing the working principles of Nebu~Flow technology. Inset shows confinement of liquid within the microstructure, which controls the largest capillary wave on the surface of liquid and generated droplet size (droplet size is proportional to capillary wavelength).
Microstructure | 400 μM | 200 μM |
MMAD (μM) | 2.2 + 0.5 | 1.0 + 0.2 |
GSD | 1.8 + 0.1 | 1.6 + 0.1 |
FPF (%) | 86.5 + 1.5 | 93.7 + 5.4 |
Table 1: Performance of Nebu~Flow technology for a wide range of formulations. The same platform was used in all experiments, without further modification. Characterisation carried out by an Andersen cascade impactor at 30 L/min. The concentrations of placebo formulation models were measured by addition of Allura Red dye.
The Nebu~Flow technology controls the aerosol droplet size by confining the liquid and surface waves in an array of microstructures.20 Nebu~Flow’s work has shown that the aerosol droplet size is proportional to the capillary wavelength on the surface-actuated liquid. Using a microstructure prevents formation of large capillary waves and consequent large droplets, working as a low pass filter (Figure 2). The Nebu~Flow technology controls the aerosol droplet size by employing microstructures in the range of hundreds of micrometres. This technology can generate aerosols with mass median aerosol diameters (MMADs) well below 5 μm, enhancing the aerosol drug delivery and increasing the fine particle fraction (Figure 3). For example, a salbutamol solution for nebulisation was delivered from microstructure arrays of 200 and 400 mm in diameter, resulting in an approximate doubling of droplet size (Table 1). This approach also has the potential to tailor the droplet size through the choice of different driving parameters. Furthermore, the technology can generate aerosols within milliseconds, providing a great platform for breath-actuated nebulisers. Additionally, the large microstructures cannot be easily blocked and can be easily cleaned, making it an easier platform to maintain.
Solution | MMAD (μm) |
GSD (μm) |
FPF (%) |
Flow rate (μL/min) |
Viscosity (cP) |
Surface tension (mN/m) |
|
Surrogate formulation models | 1% Sodium sulfate | 1.1 | 1.8 | 73.6 | 200 | 1.1 | 69 |
1% Sodium chloride | 1.4 | 1.9 | 83.1 | 200 | 1.0 | 72 | |
50% (v/v) Ethanol | 1.6 | 1.8 | 81.3 | 170 | 2.0 | 27 | |
2.5% SDS | 0.9 | 2.9 | 53.5 | 320 | 1.3 | 31 | |
2 % Tween 80 | 1.9 | 2.2 | 77.5 | 100 | 1.2 | 33 | |
10% PEG 400 | 2.4 | 1.6 | 44.3 | 100 | 1.56 | 55.7 | |
10% Glycerin | 2.0 | 1.8 | 45.6 | 120 | 1.4 | 50 | |
2% Dextran | 2.7 | 3.3 | 26.6 | 60 | 1.66 | 68.5 | |
Generic drugs | Amikacin 50 mg/mL | 1.3 | 2.0 | 42.7 | 90 | N/A | N/A |
Tobramycin 40 mg/mL | 1.1 | 1.7 | 83.7 | 210 | N/A | N/A | |
Ipratropium bromide 0.25 mg/mL |
1.4 | 1.8 | 88.1 | 100 | N/A | N/A | |
Ipratropium bromide 0.25 mg/mL |
1.7 | 1.9 | 83.7 | 210 | N/A | N/A | |
Salbutamol 2 mg/mL | 1.6 | 2.0 | 84.4 | 300 | N/A | N/A |
Table2: Control of salbutamol droplet size in a Nebu~Flow, using microstructures with sizes of 400 and 200 μm. MMAD: mass median aerodynamic diameter; GSD: geometric standard deviation; FPF: fine particle fraction as percentage emitted dose; SDS: sodium dodecyl sulfate. Characterisation carried out by an Andersen cascade impactor at 30 L/min.
Nebu~Flow technology has been used successfully to nebulise a range of placebo and commercially available formulation types with a wide range of physical properties, including those with very low surface tension (<30 mN/m) and high viscosity (2 cP), as shown in Table 2. Nonetheless, while this is a broader range of physical properties than mesh nebulisers can accommodate, there is still some dependency of output rate on these physical properties. However, in these studies, the Nebu~Flow technology was able to generate droplet sizes around half of those achieved by conventional technologies (Figure 3), indicating the rate of individual droplet creation is an order of magnitude greater.
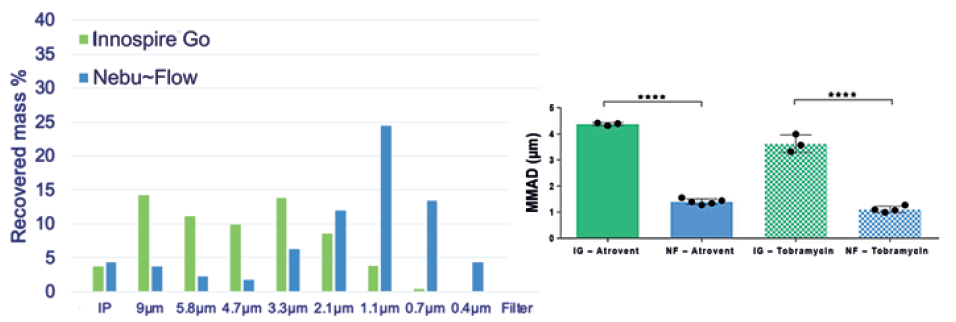
Figure 3: Cascade impaction measurement comparison of aerosols generated by Nebu~Flow (NF) and InnospireGo (IG) mesh nebuliser. A representative aerosol distribution of Atrovent (250 μg/mL ipratropium bromide) nebulisation with both Nebu~Flow and InnospireGo (left); MMAD of aerosols generated using Atrovent and Tobramycin (40 mg/mL) from Nebu~Flow and InnospireGo (right). Aerosols from Nebu~Flow have smaller sizes with MMAD in range of 1 μm, ideal for deposition in the alveolar region.
Beyond this, one of the major advantages of the Nebu~Flow technology is its ability to aerosolise biological entities without denaturing them. Acu-Flow has tested this with a range of biologics, including Herring sperm DNA and siRNA.22
SUMMARY
Inhalation drug delivery is the primary route for treatment of respiratory disorders and also shows a great potential for unlocking needle-free systemic drug delivery, while reducing side effects. The main challenges of this route are the technical limitations of current inhalation technologies; for example, the development of inhalable biologics has been delayed due to the lack of optimal devices for delivery.
Nebu~Flow technology provides a platform that can deliver a wide range of formulations with different physical properties, as well as the ability to deliver fragile biologics. The further ability of the technology to control the aerosol droplet size for deep lung deposition, with an MMAD in the range of 1 μm, can provide significant improvement for efficient inhalation drug delivery, as well as unlocking systemic drug delivery via deposition of drugs in the alveolar region. A combination of technical capabilities and patent protection makes Nebu~Flow an outstanding platform for the development of tailored drug-nebuliser products.
REFERENCES
- James SL et al, “Global, Regional, and National Incidence, Prevalence, and Years Lived with Disability for 354 Diseases and Injuries for 195 Countries and Territories, 1990–2017: A Systematic Analysis for the Global Burden of Disease Study 2017”. Lancet, 2018, Vol 392(10159), pp 1789–1858.
- Meghji J et al, “Improving Lung Health in Low-Income and Middle-Income Countries: From Challenges to Solutions”. Lancet, 2021, Vol 397(10277), pp 928–940.
- Levine S et al, “The Global Impact of Respiratory Disease – 3rd Edition”. Forum of International Respiratory Societies report, Oct 2021.
- Darquenne C, “Deposition Mechanisms”. J Aerosol Med Pulm Drug Deliv, 2020, Vol 33(4), pp 181–185.
- Martin AR, “Regional Deposition: Targeting”. J Aerosol Med Pulm Drug Deliv, 2021, Vol 34(1), pp 1–10.
- Gurbel PA et al, “First In-Human Experience With Inhaled Acetylsalicylic Acid for Immediate Platelet Inhibition: Comparison With Chewed and Swallowed Acetylsalicylic Acid”. Circulation, 2020, Vol 142(13), pp 1305–1307.
- Laube BL, “The Expanding Role of Aerosols in Systemic Drug Delivery, Gene Therapy and Vaccination: An Update”. Transl Respir Med, 2014, Vol 2, p 3.
- Liang CY et al, “Misuse of Inhalers Among COPD Patients in a Community Hospital in Taiwan”. Int J Chron Obstruct Pulmon Dis, 2018, Vol 13, pp 1309–1316.
- Price D et al, “Factors Associated with Appropriate Inhaler Use in Patients with COPD – Lessons from the REAL Survey”. Int J Chron Obstuct Pulmon Dis, 2018, Vol 13, pp 695–702.
- Pritchard JN, Nazarzadeh E, “Next Generation Nebulized Therapy: Opportunities for New Treatments and Devices”. Respiratory Drug Delivery 2022, Vol 1, pp 103–112.
- Pritchard JN et al, “Mesh Nebulizers Have Become the First Choice for New Nebulized Pharmaceutical Drug Developments”. Ther Deliv, 2018, Vol 9(2), pp 121–136.
- Lentz YK, “Effect of Jet Nebulization on DNA: Identifying the Dominant Degradation Mechanism and Mitigation Methods”. J Aerosol Sci, 2005, Vol 36(8), pp 973–990.
- Smith EC, Denyer J, Kendrick AH, “Comparison of Twenty Three Nebulizer/Compressor Combinations for Domiciliary Use”. Eur Repir J, 1995, Vol 8(7), pp 1214–1221.
- Elphick M et al, “Factors to Consider When Selecting a Nebulizer for a New Inhaled Drug Product Development Program”. Expert Opin Drug Deliv, 2015, Vol 12(8), pp 1375–1387.
- Clark AR, “Essentials for Aerosol Delivery to Term and Pre-Term Infants”. Ann Transl Med, 2021, Vol 9(7), p 594.
- Pritchard JN, “Nebulized Drug Delivery in Respiratory Medicine: What Does the Future Hold?”. Ther Deliv, 2017, Vol 8(6), pp 391–399.
- Nazarzadeh E et al, “Confinement of Surface Waves at the Air-Water Interface to Control Aerosol Size and Dispersity”. Phys Fluids, 2017, Vol 29, Article 112105.
- Kurosawa M et al, “Surface Acoustic Wave Atomizer”. Sens Actuator A Phys, 1995, Vol 50(1–2), pp 69–74.
- Rajapaksa AE et al, “Effective Pulmonary Delivery of an Aerosolized Plasmid DNA Vaccine via Surface Acoustic Wave Nebulization”. Respir Res, 2014, Vol 15(1), p 60.
- Rezk AR, Tan JK, Yeo LY, “HYbriD Resonant Acoustics (HYDRA)”. Adv Mater, 2016, Vol 28(10), pp 1970–1975.
- Rajapaksa AE et al, “Pulmonary Deposition of Radionucleotide-Labeled Palivizumab: Proof-of-Concept Study”. Front Pharmacol, 2020, Vol 11, p 1291.
- King X et al, “Ultrasonic Surface Acoustic Wave Platform for Targeted Pulmonary Delivery of Nano Drug Vehicles”. 2019 IEEE IUS, 2019, pp 699–701.
Previous article
A ROADMAP FOR DRUG-NEBULISER COMBINATION PRODUCT DEVELOPMENTNext article
STEPS TO SUCCESS IN NOSE-TO-BRAIN DRUG DELIVERY