To Issue 140
Citation: Kippax P, “Solving Complex Formulation and Delivery Challenges for Intranasal Drugs”. ONdrugDelivery, Issue 140 (Nov 2022), pp 18–22.
Paul Kippax discusses the analytical techniques used for in vitro characterisation of nasal sprays, and how their use can accelerate and de-risk both innovator and generic nasal drug development.
Given the choice, many patients would prefer to avoid injections or other similarly invasive methods of delivering medicines. One route offering an alternative approach for a rapidly expanding range of treatments is nasal administration. As well as addressing any fear of needles and the risk of injection pain, nasal administration can enable the more rapid onset of therapeutic effects. For example, its use in vaccine delivery means less stress for the patient, reduced training requirements for administration, the potential for more relevant immune responses in the mucosal tissues where viruses enter the body and possible cold chain advantages compared with current vaccine products.
“Nasal drug products are classified as complex pharmaceutical formulations because of the interdependence of the formulation and the delivery device.”
By no means can all drugs be administered this way, but intranasal delivery continues to move on from its origins in administering locally acting substances to playing an important role in a diverse range of systemic (as well as local) treatments.1–4 Today, ground breaking work on vaccines, prophylactics and therapeutic compounds for SARS-CoV-2 is putting the intranasal route firmly in the spotlight as researchers seek the most effective means of delivering these medicines. With nasal drug delivery on the rise, this article reviews some of the key challenges scientists face in developing the complex formulations involved, and also examines the role of physicochemical analysis in defining appropriate solutions.
NASAL DELIVERY – AN EVOLVING FORMAT
Locally acting decongestants and treatments for allergic rhinitis were among the first modern pharmaceutical formulations developed specifically for nasal delivery.5 More recently, analgesics, anti-depressants, hormone treatments and other systemically acting drugs have joined their ranks.
The nasal cavity’s large surface area and high blood flow for absorption enable direct application and rapid relief when using locally acting pharmaceuticals.6,7 For systemic therapies, nasal delivery provides a non-invasive route that allows rapid absorption while also bypassing the gastrointestinal tract. Additionally, the possibility of direct nose-to-brain delivery opens the way for drugs unable to cross the blood-brain barrier to rapidly enter the central nervous system.8
The SARS-CoV-2 pandemic has heightened interest in nasal delivery. As investigators continue to explore this route for coronavirus-related vaccines,9 as well as other therapies and prophylactic treatments,10 pharmaceutical scientists are under increasing pressure to manage the formulation challenges effectively.
THE FUNDAMENTALS AND COMPLEXITY OF NASAL SPRAYS
While dry powder formulations are used in some applications, drug formulations for nasal delivery generally consist of an API dissolved or suspended in an aqueous formulation. The product is delivered via an aerosolisation device, such as a metered dose spray pump, to ensure easy and well-controlled administration.
Nasal drug products are classified (alongside orally inhaled preparations)as complex pharmaceutical formulations because of the interdependence of the formulation and the delivery device. Successful development of a nasal drug product requires both an effective formulation and a detailed knowledge of how to deliver it consistently using a complex device. Understanding and managing the impact of both elements is essential, whether you are developing innovator or generic drugs.
The most important aspects of nasal sprays are that they must enable delivery of the drug substance to the target tissues and achieve the required bioavailability in the patient. Optimising the formulation for bioavailability and the device for delivery requires a complete understanding of how the two interact, and the impact of those interactions on delivery and efficacy. For example, the design and geometry of a spray nozzle affects the stress on the suspension and therefore impacts the resulting spray droplet size and distribution. This, in turn, influences where and how the droplets deposit in the patient’s nose and, as a result, the drug’s bioavailability and speed of action. Likewise, the addition of an excipient to improve nasal retention time can change the formulation viscosity and alter the pumping efficiency and “sprayability” of the product, with consequences for deposition and bioavailability.
Delivered droplet size is a critical quality attribute for nasal products, one that directly affects clinical efficacy. Correlation between the spray droplet size and in vivo deposition behaviour for nasal products means that particle size distribution (PSD) measurement is essential during development, manufacture and quality control. Equally, the PSD of the API within the dispersed aerosol is important in defining the efficacy of drug delivery, as well as overall bioavailability.
EXPLORING NASAL DRUG DEVELOPMENT CHALLENGES
A particular challenge when developing nasal products is that systemic drug concentration is not necessarily a good indicator of local drug concentration and therapeutic benefit. Consequently, traditional pharmacokinetic assessments of blood plasma concentration may not be relevant in determining bioavailability and equivalence. To help mitigate this, developers increasingly use in vitro bioequivalence (IVBE) testing – the process of using in vitro techniques to assess a test product’s bioavailability and bioequivalence compared with the corresponding reference listed drug – to optimise and accelerate development. Underlying this trend is a recognition of the importance of the formulation microstructure in controlling drug delivery and release.
In recent years, regulators have released specific guidance on the assessment of IVBE in nasal drug products.11 Although this has predominantly been targeted towards the rapid development of generics, it also highlights the critical material attributes (CMAs) that are important for device and formulation optimisation for innovator product research and development. Consequently, data from in vitro methods is helping to drive both generic and innovator product development.
Physicochemical characterisation plays a crucial role in measuring properties that are predictive of bioavailability and answering key questions about the delivery of nasal sprays. For example, “What is the spray and where does it go?” requires an understanding of droplet size, plume geometry and spray angle, whereas “How stable is the formulation?” demands knowledge of the API particle size and formulation viscosity. Among the physicochemical characterisation techniques used are laser diffraction particle size analysis for droplet size measurement, the recently developed morphologically directed Raman spectroscopy (MDRS) for API characterisation and size exclusion chromatography (SEC) to elucidate the properties of polymer excipients.
UNDERSTANDING DROPLET ATOMISATION DURING NASAL SPRAY DELIVERY
Controlling droplet size in nasal sprays is crucial to successful in vivo drug deposition. Nasopharyngeal deposition typically requires droplets in the range 20–120 μm. Smaller particles are likely to be deeply inhaled, while larger droplets will remain near the outside of the nose. Droplet size is a function of the formulation’s physical properties (most notably viscosity), along with the mechanism and geometry of the delivery device. By manipulating formulation and device variables, developers can engineer the production of appropriately sized droplets.
Laser diffraction is the recommended technique for determining droplet size distribution in nasal sprays. Its rapid measurement capability delivers valuable information during a high-speed spray event, from device actuation right through development and then termination of the spray plume. As the following examples illustrate, understanding droplet size supports many aspects of drug and device formulation performance. It can even indicate how a product might perform in different hands. Droplet size distribution data is essential for comparing formulations in bioequivalence studies.
Figure 1 shows a set of droplet size distribution measurements that can help elucidate atomisation during a nasal spray event. Droplet size (y-axis) was measured using a laser diffraction particle size analyser designed specifically for sprays (Spraytec – Malvern Panalytical). Shown versus time are the Dv10 (the size below which 10% of the particles in the sample fall), Dv50 (the median droplet size) and Dv90 (below which 90% of particles fall), as well as the transmission level (right-hand axis). The lower the transmission, the higher the concentration of the aerosol, allowing observation of spray plume development through the droplet measurement zone. Concentration is low as actuation begins, then the main part of the spray plume moves through the measurement zone. Finally, as actuation stops, transmission rises. In the formation phase at the beginning, the droplet size is briefly relatively large, then drops in the stable phase where the plume is fully developed. When actuation ends, the droplet size increases significantly as atomisation moves out of control.
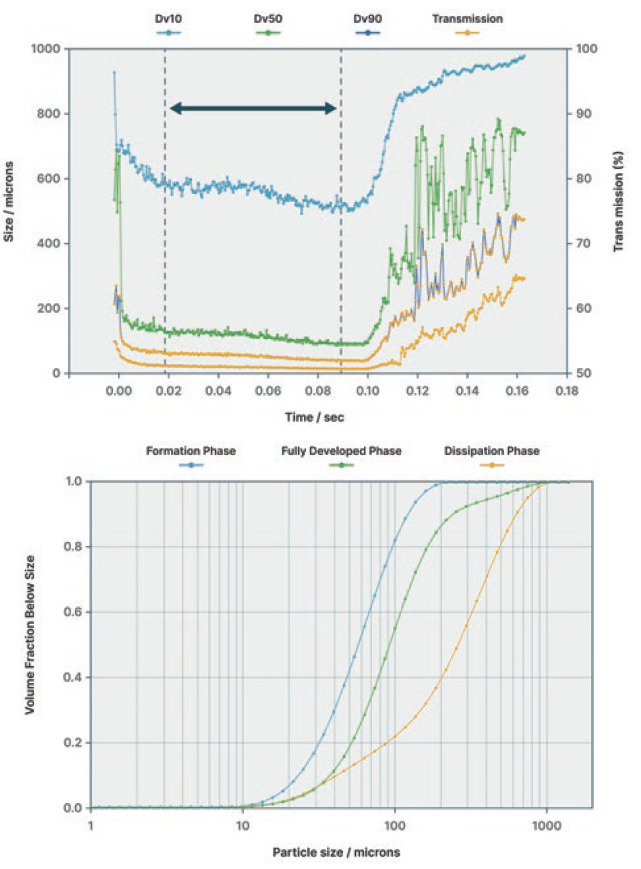
Figure 1: Understanding droplet atomisation during nasal spray delivery.
Bioequivalence studies require isolation of the fully developed stage – the stage during which deposition in the nose is most likely to occur in the most controlled way – for comparison between products. Instrument software enables isolation of all phases, making this comparison straightforward.
Real-time measurement of droplet size distribution also helps quantify how variations in CMAs, such as formulation viscosity (rheology) or nozzle geometry, impact performance. Figure 2 compares the median droplet size delivered by a particular nasal spray device for formulations of different viscosities. At concentrations of 0%, 0.25% and 0.5% polyvinylpyrrolidone (PVP), the formation, fully developed and dissipation phases are all present. At higher PVP concentrations (higher viscosities), actuation is less successful, droplet size is larger and less stable, and the fully developed phase is less well defined. These profiles give an indication of how the formulation and device work together and point to where changes might be required. As shown on the right of Figure 2, the droplet size data links to other IVBE parameters, such as spray plume geometry (SprayVIEW – Proveris Scientific), which in this case confirms that the device fails to atomise the high PVP concentration liquids.
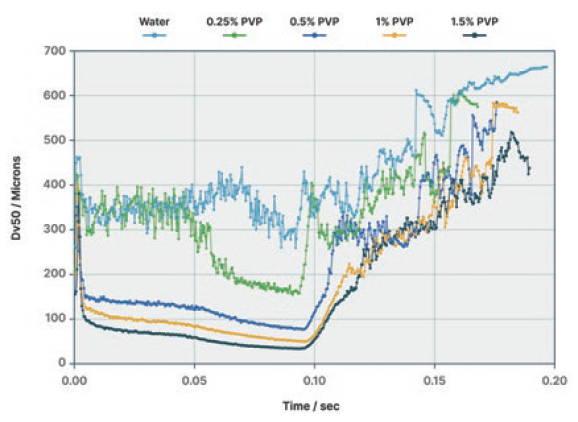
Figure 2: Linking rheology to droplet atomisation during nasal spray delivery.
Figure 3 shows results of using the same PVP formulations but a different delivery device, one which has a mechanism for storing energy that is released at the end of pump actuation. This changes the nature of the fully developed phase, giving a more stable droplet size. Although the Dv50 increases with increasing viscosity, atomisation of the liquid, even at the highest concentration, is controlled, building evidence to help match the formulation with the most appropriate delivery device.
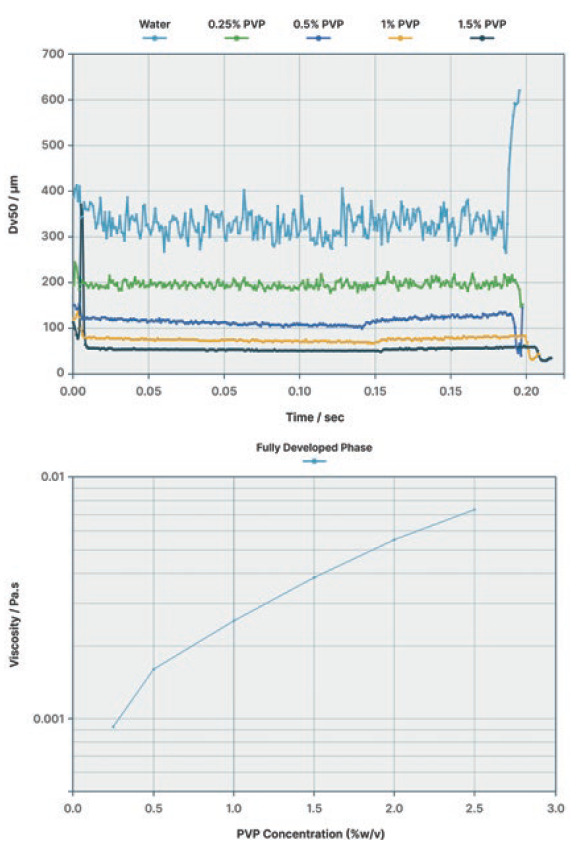
Figure 3: Linking droplet size to device selection.
USING API PARTICLE SIZE FOR INSIGHTS INTO BIOAVAILABILITY
API particle size gives information on the dissolution rate and the likely bioavailability of the drug. However, discriminating between API and excipient particles in nasal spray suspensions is a significant challenge. Component-specific particle size measurement using MDRS, a microscopy-based technique that combines automated imaging technology with Raman spectroscopy, delivers particle shape, size and component-specific chemical identification data.
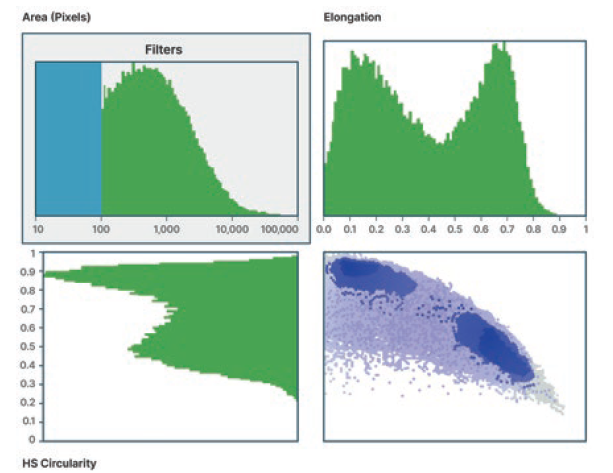
Figure 4: Selecting classification parameters for API and excipient particles.
In the MDRS workflow, particle size and shape analysis using the Morphologi 4-ID (Malvern Panalytical) helps separate excipient and API particles, as shown in Figure 4. Development of this particle size and shape classification enables targeting of the system’s Raman analysis towards the groups likely to contain the API, that being particles with a low elongation ratio in this case. Raman spectroscopy is then applied to discriminate API from excipient within this particle set. Particles are classified by comparison with reference spectra, which enables the determination of API particle size distribution and comparisons with other products (Figure 5).
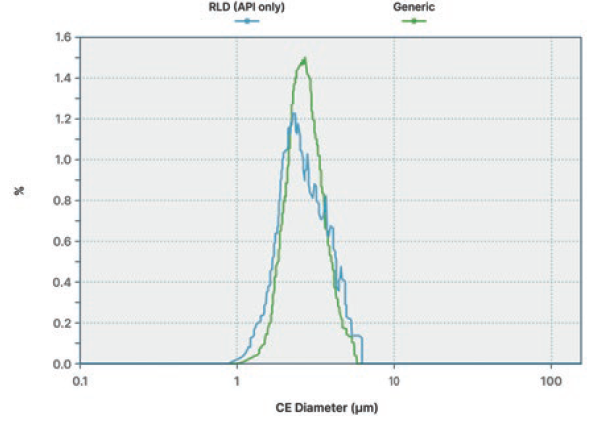
Figure 5: API component-specific particle size distribution.
Third-party studies have demonstrated the correlation between MDRS data and dissolution rates and indicate that MDRS is relevant for assessing bioequivalence in nasal sprays.12 The same studies also examine the relationship between rheological performance and spray droplet size distribution measured by laser diffraction, again demonstrating a good correlation.
GETTING A BETTER HANDLE ON EXCIPIENT BEHAVIOUR
The physicochemical properties of polymeric excipients can directly affect the clinical efficacy, safety and quality of finished pharmaceutical products, and are routinely identified as CMAs. Excipient choices make a critical difference to nasal spray performance, with polymers often used to modulate viscosity. As already discussed, this can directly impact spray droplet size distribution and affect the ultimate deposition and bioavailability of the API.
The molecular weight, molecular weight distribution and structural characteristics of such excipients define their behaviour. Here, SEC is an established technique for the measurement of these properties and plays an important role in pharmaceutical formulation. The use of multiple detectors, including for light scattering, viscosity and concentration, enables the measurement of absolute molecular weight, molecular size and intrinsic viscosity, generating information on the macromolecular structure and branching. Multiple detector systems (such as OMNISEC – Malvern Panalytical) deliver the high sensitivity needed for precise differentiation of excipient grades and offer automated and highly repeatable measurement, for efficient, high-productivity analysis.
MORE THAN A SHOT IN THE ARM
The growing appeal of nasal delivery for a widening range of pharmaceuticals puts pressure on developers to overcome the challenges of these complex formulations where drug formulation and device development must proceed hand in hand. Recognising the inseparability of these processes is fundamental to putting strategies in place to de-risk and accelerate product development.
Regulatory guidance for demonstrating IVBE in nasal spray products is benefiting developers of both generics and innovator products. It highlights the importance of formulation microstructure in controlling drug delivery and release, and points to the role of physicochemical characterisation techniques in measuring and understanding changes. Methods such as laser diffraction particle sizing and MDRS are helping developers understand product behaviour in ways that accelerate progress and support success. The use of these techniques within a range of analytical approaches makes formulation optimisation more effective, which ultimately helps to deliver a greater choice of highly effective and easier to administer pharmaceutical products.
REFERENCES
- Laffleur F, Bauer B, “Progress in Nasal Drug Delivery Systems”. Int J Pharm, 2021, Vol 607, Article 120994.
- Suman J, “How Evolving Patient Needs Have Fuelled the Development of Nasal Drug Delivery”. ONdrugDelivery, Issue 119 (Apr-May 2021), pp 18–22.
- Fortuna A, Schindowski K, Sonvico F, “Intranasal Drug Delivery: Challenges and Opportunities”. Front Pharmacol, Mar 2022.
- Klein M-C, Bilstein A, Kraus R, “Nose-to-Brain Drug Administration and a Novel Approach with an Old Familiar”. ONdrugDelivery, Issue 131 (Apr 2022), pp 22–24.
- Suman JD, “Nasal Drug Delivery: Past, Present and Future Perspectives”. Inhalation, Jan 2020.
- Verma S, Tyagi Y, Tangri P, “Review Article on Nasal Drug Delivery System”. IJSDR, 2021, Vol 6(2), pp 100–107.
- Keller LA, Merkel O, Popp A, “Intranasal Drug Delivery: Opportunities and Toxicologic Challenges During Drug Development”. Drug Deliv Transl Res, 2022, Vol 12, pp 735–757.
- Wang Z et al, “Nose-to-Brain Delivery”. J Pharmacol Exp Ther, 2019, Vol 370(3), pp 593–601.
- Alu A et al, “Intranasal COVID-19 Vaccines: From Bench to Bed”. eBioMedicine, 2022, Vol 76, Article 103841.
- “Nasal Spray to Fight COVID-19 Heads to Clinical Trial”. University of Melbourne, Dec 2021.
- “Bioavailability and Bioequivalence Studies for Nasal Aerosols and Nasal Sprays for Local Action”. FDA Guidance for Industry, Apr 2003.
- Shur J et al, “Characterizing Nasal Suspensions for Regulatory and Scientific Purposes”. Respiratory Drug Delivery 2016, 2016, Vol 1, pp 185–192.