Citation: Volmer J, Brown M, Drummond J, “The Challenges and Opportunities of Drug Delivery Through a Complex Barrier”. ONdrugDelivery Magazine, Issue 94 (Jan 2019), pp 6-9.
Jon Volmer, Marc Brown and Jeremy Drummond discuss the development and use of advanced ex vivo and in vitro models for testing new ocular medicines to meet a growing unmet need.
INTRODUCTION
The prevention and treatment of diseases of the eye represents a US$23 billion (£18.2 billion) annual market globally.1 The major impact eye health and vision make on quality of life means that this is a rewarding area for development of any drug products that meet a medical need. Modern lifestyles and an ageing population are also bringing the need for solutions to ocular diseases, such as dry eye disease (DED), to the attention of the ophthalmology community. The pharmaceutical industry has responded to this and there are currently over 2,500 clinical trials underway related to ocular drug products.2 This number is being boosted by a greater understanding of basic biology in other therapeutic areas, which is uncovering overlaps with certain pathways and targets in the eye, especially in immunology and inflammation.
“To be able to formulate a topical eye product successfully, it is fundamental to understand the complex, multilayered nature of the surface of the eye…”
As a distinct organ with easy access, the eye is ideal for direct drug delivery. This can be achieved topically or by either intravitreal or trans-scleral injection. From the patient perspective, any drug with the possibility of being delivered topically will be preferable to an injection that must be performed by an appropriate healthcare professional (HCP).
Ocular delivery presents both unique challenges and opportunities due to the eye’s structure and function. To be able to formulate a topical eye product successfully, it is fundamental to understand the complex, multilayered nature of the surface of the eye. This understanding can be greatly enhanced by an ability to create relevant and meaningful models of this unique barrier in order to select the appropriate drugs and optimise formulations within the laboratory. Such knowledge gives a product the best chance of providing positive health benefits to the patient.
THE DRUG DELIVERY CHALLENGE
An understanding of the structure of the eye surface barrier is crucial to understanding how a drug is likely to reach its site of action (Figure 1).
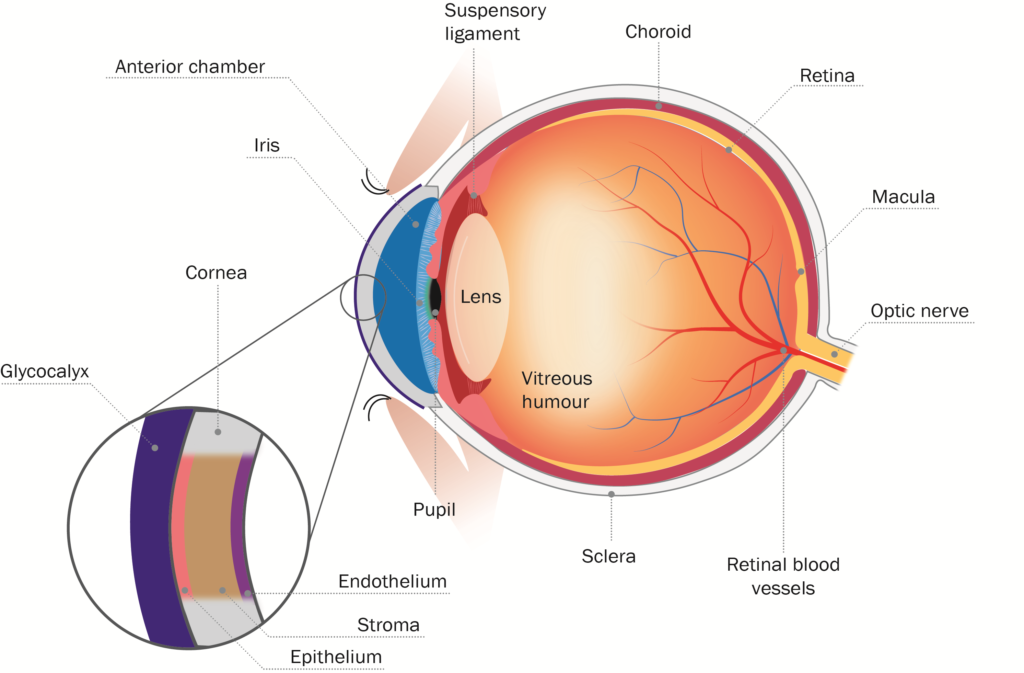
Figure 1: Schematic diagram of eye and corneal barrier.
The first barrier on the surface of the eye comprises the glycocalyx, which are long chain molecules that help hold mucin to the corneal surface. Formed by corneal cells, glycocalyx migrate out from the surface of the corneal microvilli to form a hydrophilic network that holds mucin on the ocular surface. The glycocalyx is a well-ordered gel layer, extending 200–500 nm above the corneal epithelium.3 Although it is thought that the primary function of this layer is to maintain hydration and lubrication of the corneal surface, it also presents a significant barrier to drug delivery, as water binding and displacement by the glycocalyx can have significant impact on effective concentrations and gradients. The glycocalyx can also potentially be exploited as a depot platform to retaining drug near the corneal epithelium.
“For MedPharm and its clients, in vitro and ex vivo models have proven themselves invaluable for optimising topical drug formulations and de-risking development programmes…”
Below the glycocalyx lies the cornea itself, comprising three distinct layers, the epithelium, the stroma and the endothelium. The epithelium presents a significant barrier because of its lipophilic nature and extensive tight junctions, which closely link the cells and therefore leave little space for diffusion between them. Corneal epithelial cells also express an array of ATP-binding cassette efflux transporter pumps. These pumps actively remove lipophilic molecules, organic anions and conjugated compounds from the cytoplasm of corneal epithelial cells. They represent one of the most active elements of the corneal barrier and as such ideally need to be avoided when optimising drug delivery. Corneal epithelial cells have also been shown to express an array of solute-linked carrier (SLC) influx transporter pumps, which actively bring in nutrients and signalling molecules, making them a tempting target for drug delivery to the corneal epithelium.4 There is also evidence that corneal epithelial cells have the potential to phagocytose particulates from the tear film.5
Below the corneal epithelium is the stroma, which is comprised of highly structured collagen lamellae, several hundred micrometres thick (comprising almost 90% of the thickness of the cornea) and highly hydrophilic. Whilst lipophilic drugs are preferable for permeation through the corneal epithelium, hydrophilic drugs are preferred for permeation through the stroma. This can be exploited in drug structure optimisation, depending on which compartment is being targeted.
The final layer of the cornea is the endothelial layer, separating the stroma from the anterior segment of the eye. The leaky nature of cell-cell interactions at the endothelium means it represents minimal function as a barrier.
The eye, in a similar way to any external surface on the body, has evolved to prevent intrusion by foreign molecules and particles. However, an influx of inflammatory cells and vascularisation, as seen with other barriers such as the skin, are not an effective early response mechanism for the eye due to the fact that the cornea must remain transparent. Thus, the compact surface barrier of the cornea and sclera is complimented with a protective stream of tears and the regular sweeping of the eyelid. This makes topical drug delivery to the eye a significant challenge.
When a formulation is applied to the surface of the eye, clearance of the product is immediately initiated. The normal tear volume on the surface of the human eye is approximately 7 μL, and the exposed surface of the eye can typically hold a maximum of about 30 μL before overflowing. This limits any useful topical application to around 23 μL. Tear volume usually reverts to normal within 2–3 minutes. In addition, the blink response can amplify this clearance dramatically. It can result in a total contact time of the drug with the absorptive surface of the eye of five minutes or less.6
This leaves much room for improvement through the selection of drugs with permeation properties specific to the barriers present in the eye and the design and development of optimised bio-adhesive formulations. Appropriate in vitro and ex vivo models are therefore a critical tool in topical product development for the eye.
EFFECTIVE MODELS FOR DRUG DELIVERY TO THE EYE
For MedPharm and its clients, in vitro and ex vivo models have proven themselves invaluable for optimising topical drug formulations and de-risking development programmes because they provide a strong indicator of the likely performance in the clinic, and models of the eye continue to gain in sophistication.
Prior to any drug penetration or permeation taking place, it is important to maximise the opportunity for a formulated drug to get to the surface of the eye and bind against the flow of tears. For many years, MedPharm has used ex vivo models in which the level of adhesion to corneal tissue is measured whilst the surface is perfused with artificial tear fluid at a defined rate. In MedPharm’s testing approach, the drug release from the formulation and the residency time of the formulation on the eye are maximised in parallel to maintaining chemical and physical drug stability, thus providing the best chance of success. In order to mitigate the risk of failure, a wide range of excipients (including but not limited to penetration enhancers and bio-adhesive agents) need to be tested in numerous formulations.
“The combination of tissue culture and human/animal eye models for the evaluation and optimisation of drug/formulation retention, drug permeation and penetration, and disease activity provides a powerful toolkit for ocular drug product development…”
These in vitro and ex vivo models help to characterise and optimise formulations in pursuit of increasing their residency time in the presence of tear fluid, whilst limiting vision impairment, in a cost-effective and efficient manner. This also negates the need for performing relatively expensive in vivo studies in animals at an early stage. These models can be used to screen larger numbers of formulations than animal studies. Typically, MedPharm develops the adhesion models for a specific drug compound using the appropriate ex vivo animal eyes. The use of human corneal tissue, sourced from cadavers, is restricted to in vitro permeation and penetration testing and disease models.
MedPharm use corneal tissue for assessing vehicle and formulation effects on the penetration and permeation of a drug into and across the cornea using modified static and validated diffusion cells. Animal corneal tissue is used during method development and then final experiments are carried out using human corneal tissue. These experiments give a good idea of the likely challenges for a drug’s delivery and any concentration build up in a particular layer of the cornea can be identified.
Whether from humans or animals, these models have the benefit of using real corneal tissue, possessing all of the gross anatomical characteristics. Care must be taken in interpreting results from these studies, as some of the barrier function in the cornea is due to active processes. Treatment during harvest, storage, transportation and processing of the cornea is likely to result in damage or degradation of the tight junctions of the corneal epithelium. While the integrity of the tissue can be screened prior to experimentation by measuring transepithelial resistance, other less easily detected damage may also occur. The glycocalyx can be sloughed or damaged and efflux/influx transporters will be functionally lost. When using models of this type MedPharm recognises the need for fast and careful preparation and also careful interpretation of results in the knowledge that some drugs may behave differently in more viable eye tissue.
For obvious reasons, there is not a readily available supply of fresh, healthy eye tissue. Biologists at MedPharm are increasingly relying on tissue culture models based on primary corneal epithelial cells from healthy rabbits, as well as commercially available human sources, to form a functional, well differentiated epithelium as an appropriate replacement.
For these models, the cells are grown in transwells (Figure 2) on a membrane supported on the surface of cell culture media. Cells are in contact with media on the basolateral side and exposed to air on the apical side. As in many epithelial tissues, this polarised culture condition promotes the formation of tight junctions, production of appropriate mucins or other surface proteins, and the establishment of a barrier very similar to the natural barrier offered by an intact corneal epithelium. Cells grown in this fashion exhibit transepithelial resistance similar to that of native corneal tissue, which strongly suggests that functional tight junctions are present.7 Furthermore, these models have the potential to express a functional glycocalyx and an appropriate efflux/influx transporter system. Additionally, expression of the appropriate genes has been observed at the transcript level. These models can also be used to assess drug activity by upregulating key disease biomarkers. They offer the formulator the key benefit of being a cost-effective and readily available way of optimising a product by allowing the relatively rapid testing of multiple drugs and formulations.

Figure 2: Diagram of a transwell.
There are also models available for corneal tissue based on immortalised human corneal epithelial cells. MedPharm’s preference is not to use these models because they can undergo significant metabolic changes through the transformation process needed to maintain long-term viability. These changes can result in a significant depletion in integrity as measured by trans-epithelial resistivity compared with primary cell cultures, which will have a significant impact on the ability of a drug to permeate and penetrate the tissue.
More recently, MedPharm has developed a set of tissue culture and human/animal eye models for various diseases. These enable its clients to screen their drugs and/or formulations against eye conditions such as DED, infections and inflammatory conditions (for example conjunctivitis, keratitis, uveitis and blepharitis). These models are disease and drug specific and allow for comparison of the activity a new drug/formulation with those already marketed.
MedPharm continues to develop novel eye delivery models to support its customers’ ocular product developments. Changes in media and culture conditions continue to lead to even greater alignment to the native eye tissue. The combination of tissue culture and human/animal eye models for the evaluation and optimisation of drug/formulation retention, drug permeation and penetration, and disease activity provides a powerful toolkit for ocular drug product development. The next step is to combine these more sophisticated biological models with physical components, such as tissue clearance in a single eye model.
CONCLUSION
The unique nature of the epithelial barrier in the eye presents a significant challenge in the development of effective treatments for ocular diseases. The development and use of increasingly relevant in vitro and ex vivo models reduces the risks associated with this challenge. These models can be used as a cost-effective screen for selecting drug candidates and identifying the optimal formulation prior to any significant investment in their development. They offer significant advantages over in vivo animal models when there is a need to test large numbers of formulations. The demand for ocular pharmaceuticals to meet current unmet medical needs will undoubtedly continue to increase, and these models will play a key role in reducing the risks, costs and timelines associated with any such development.
REFERENCES
- “Global Ophthalmic Drugs Market Forecast 2017-2027”. visiongain, Jun 2017.
- Analysis of BioPharm Insight database, Dec 2018.
- Argüeso P, “Glycobiology of the ocular surface: mucins and lectins”. Jpn J Ophthalmol, 2013, Vol 57(2), pp 150–155.
- Gaudana R et al, “Ocular drug delivery”. AAPS J, 2010, Vol 12(3), pp 348–360.
- Niederkorn JY, Peeler JS, Mellon J, “Phagocytosis of particulate antigens by corneal epithelial cells stimulates interleukin-1 secretion and migration of Langerhans cells into the central cornea”. Reg Immunol, 1989, Vol 2(2), pp 83–90.
- Ghate D, Edelhauser HF, “Barriers to glaucoma drug delivery”. J Glaucoma, 2008, Vol 17(2), pp 147–156.
- Barar J et al, “Ocular Drug Delivery; Impact of In Vitro Cell Culture Models”. J Ophthalmic Vis Res, 2009, Vol 4(4), pp 238–252.