To Issue 145
Citation: Klein M-C, Bilstein A, Hoffmann C, “The Application Diversity of Metered Pump-Based Soft Mist Devices”. ONdrugDelivery, Issue 145 (Apr 2023), pp 56–60.
Marie-Christine Klein, Andreas Bilstein and Caroline Hoffmann discuss the underlying techniques involved in the use of metered pump-based soft mist devices and their application diversity.
The respiratory tract fulfils the physiological functions of vocalisation, protection against contaminants and active gas exchange between the blood and the atmosphere. Being exposed and the first line of defence, it is also a common site for infections and is subject to inflammatory events that can lead to chronic inflammatory diseases. As such, drug targeting to the distinctive parts of the respiratory tract is crucial in managing the treatment options.
“Drug targeting to the distinctive parts of the respiratory tract is crucial in managing the treatment options.”
The nose and the nasal cavities, with their angled architecture and the filter function of the epithelium, are a perfect chaperone for aerosols on their way to the lower respiratory tract. Together with the pharynx (throat), the nose and the nasal cavities create the upper airway, which in terms of inhalation is followed by the lower respiratory tract containing the larynx, the trachea, the bronchi and the lungs. The histological characteristic of each section is adapted to the respective function, which is for the so-called conducting zone (nose to terminal bronchioles) to filter and warm the air as a prerequisite for the gas exchange that takes place in the respiratory zone, with the lungs as the main respiratory organs.
The upper respiratory tract, with its nasal cavities, constitutes an interesting entry of drugs for systemic uptake and even provides a way to bypass the blood-brain barrier by using the olfactory pathway for direct targeting of brain tissue. The intranasal route can overcome the hepatic first-pass effect, which results in higher bioavailability of the drug in a lower dosing – and consequently less side effects.
The limitations of the nose-to-brain target route are mainly the small volumes (25–200 μl) that can be applied to the nasal cavity, which may limit the use to potent drugs. Furthermore, the olfactory region represents only a small surface fraction of the total cavity (5.2%), to be reached with a well-balanced droplet size. Also, the mucociliar clearance is an obstacle for drug delivery, since it generates a potent driving force from the respiratory cavity towards the larynx, limiting drug interaction time with the epithelium.
Drug targeting to the lower respiratory tract and the pulmonary tissue – the site of action for gas exchange – has the big advantage of having good pharmacokinetics for systemic-acting drugs to bypass the gastrointestinal (GI) tract with less effective absorbance. This is also the site of action for topically acting drugs specifically acting on the lung tissue. To successfully target a drug to the alveolates, the lower respiratory tract for metabolisation, many barriers built to prevent pathogen containing aerosols intruding into the lower airways need to be overcome and taken into consideration when creating a drug and choosing an application system. The mechanical barriers created by the architecture of the respiratory tract, and chemical and immunological barriers posed by the respective inhabitant cells and their secretion products, each lower the probability of successful drug bioavailability. Moreover, some clinical conditions lead to extensive mucous production or bronchoconstriction, which can increase the barriers for drug delivery.1
LARYNX AS AN INTERESTING TARGET SITE
Whereas the deep lung and the anterior nasal cavity are well-known drug targets, with many different devices on the market, the deep nasal cavity and the connection between the nasal cavity and the deep lung (trachea, larynx, voice cord) are not targeted specifically by many devices so far. The deep nasal cavity as an interesting area to be targeted for local application was described in a previous article in ONdrugDelivery.2
The larynx, as part of the conducting zone that is inevitably passed during inhalation, is also worth looking at with regard to a potential drug target for some indications. Using the larynx route for rehydration therapy and airway hygiene is a simple and potentially highly effective idea. There are studies that show that poor hydration has a negative effect on infection protection, which made affected individuals more susceptible to covid-19.3,4
“The ability of inhalers to target medication to a body part that is accessible from the outside has increased the treatment options for different highly prevalent diseases.”
The underlying mechanism for why airway dehydration has this impact is that inhaled contaminants are not filtered in an ideal way. Dehydration leads to thinning of the upper airway lining fluid, decreases the beat frequency of cilia and impairs epithelial cells. Of course, this not only holds true for SARS-CoV2 – it applies to other airborne infections as well as allergies, asthma and chronic obstructive pulmonary disease,3 as well as general air dryness exposure, as in air-conditioned rooms. Furthermore, the larynx also poses an interesting drug target for topical cancer treatment or local anaesthesia, the latter already being targeted by a spray nozzle unit (SNU)-based product, the Trachospray.5,6
DIFFERENT INHALING DEVICES FOR TARGETING THE LOWER AIRWAYS
The ability of inhalers to target medication to a body part that is accessible from the outside has increased the treatment options for different highly prevalent diseases.
Metered dose inhalers (MDIs) store the medication in pressurised form. Using propellants for aerosol production, a high velocity of 8 m/sec during a time interval of 0.1–0.4 s is achieved.7 The lung deposition overall is 10%–20%, differing with formulations and the application by the patient, which is the main drawback because of the difficulty of timing inhaler use correctly.8 The cold sensation at the back of the throat as the propellant evaporates may be destructive since the patient may stop inhaling intuitively. Nevertheless, MDIs are widespread, relatively cost effective and contain many doses. The disadvantages are being partially overcome by developments such as breath-actuated MDIs, which help to co-ordinate firing and inhaling, and MDIs with spacers that leave the dose of medication in the spacer without being blown away.8 These further developments come at the cost of a less handy design and portability.
Dry powder inhalers are breath actuated and do not need any propellants to generate the aerosol. The need for a forceful inhalation to disperse the powder is crucial for the application and, at the same time, the major drawback as it requires good understanding of the device and training of the user.
Nebulisers use either jet, ultrasound or vibrating mesh to nebulise a liquid formulation. Each aerosol generation technique comes with its own advantages and disadvantages, but the overall idea is to allow the patient to inhale during normal tidal breathing, without the need for timing the firing or breathing forcefully. This may be attractive for patients with co-ordination problems – or in emergency cases. Nebulisers have a static design, are more expensive and sometimes struggle with correct dosing and lung deposition.9
Soft mist inhalers allow a handy design and make the use of propellants unnecessary. Multidoses of a liquid formulation are stored and a slow-moving aerosol is created by a precisely engineered nozzle system. The slow velocity is the main difference compared with pressurised MDIs (pMDIs) and the idea is to give the patient enough time to co-ordinate the firing and inhaling of the aerosol through the upper and lower respiratory tract.
HUMAN FACTORS IN DRUG DEPOSITION TO THE RESPIRATORY TRACT
The above walkthrough of the different inhalers makes it clear that the operating principle differs a lot from device to device – and the patient or physician has to choose the device that best fits the abilities of the operator and the underlying disease and its treatment regimen. Training and successful handling of the device have a significant influence on the success of drug targeting.10
REQUIREMENTS FOR THE DROPLET/PARTICLE SIZES OF THE INHALING DEVICE
Besides dealing with the anatomically and cellular circumstances and the exogenous factors by handling that influence the drug deposition, the drug needs to be transformed in droplet/particle sizes that are ideal to reach their distinctive site of action.
“A device technology that allows targeting of the different areas using the same base technology has been missing so far.”
If the aerosol is entering the upper respiratory tract through the nose, small droplets <5 μm will not usually stay in the nasal cavity but will find their way to the lower respiratory tract. The higher the droplet size, the more the anterior parts of the nasal cavity will be targeted. Smaller droplets/particles have a higher chance of reaching the posterior parts of the nasal cavity, including, for example, the olfactory region, an ideal target for nose-to-brain delivery. It is not yet clear what is the best droplet size to reach the olfactory region, but there are suggestions that a diameter of ~10 μm may be favourable.
In oral inhalation, particles >5 μm mainly deposit in the oral cavity, the larynx and the trachea – i.e. the upper and conductive airways. A deep-lung deposition is reached with a particle size of 0.5–4 μm,whereas 4-5 μm sized particles deposit in the bronchi. Particles smaller than 0.5 μm are being exhaled and are not therapeutically active.11 As discussed, the different regions are targeted currently by different devices using diverse technologies to generate the particles. A device technology that allows targeting of the different areas using the same base technology has been missing so far.
SOFT MIST APPLICATOR WITH SNUS
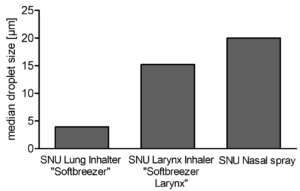
Figure 1: Median droplet sizes (μm) of inhalers or nasal spray containing different pore sizes of the SNU.
The production of aerosol by the principle of Rayleigh break-up (the generated jet breaks up automatically in droplets) inside the platform of an MDI or a nasal spray is a straightforward development that has a broad application spectrum and adaption possibilities that allow flexible therapeutic options for different target sites of the upper and lower respiratory pathways. The liquid is dispersed into droplets by mechanically pressing the liquid through a microchip with pores (SNU) and succeeds in producing a very constant particle size distribution and delivery rate. All droplets are twice the size of the hole in the chip. By changing the diameter of the hole in the spray nozzle, the droplet size can be engineered for optimal performance.12,13 For generation of the necessary force to generate the droplets, either air pressure or liquid pump technology can be used. Metered pump technology, especially when combined with the possibility of delivering preservative-free solutions, should be the ideal liquid pressure generator for a device family that profits from both the portability of a standard MDI and the generation of soft mist.
“Combining spray nozzle technology with pump-based actuation and the option of preservative free solutions enables the development of similar devices targeting specific regions of the lower and upper airways.”
On the basis of adapting the pore sizes, different targets of the respiratory tract can be tackled in an optimised manner. Figure 1 shows droplet sizes of different devices that are adjusted in terms of pore sizes and number of pores per chip. The data reflect the medium droplet sizes in micrometres and show that the adaption succeeds in producing devices with different application scenarios.
To test the different pore sizes in their real distribution of droplets among the impact sites, deposition tests in an Alberta Idealized Throat have been performed and quantified. An example measurement is given in Figure 2. The deposition in the model reflects the different distribution patterns in the oral cavity and throat dependent on the pore size of the SNU. The smaller pore size of the lung inhaler constructed to reach the deep lung achieved only 58% deposition in the oral cavity, compared with 95% deposition produced by the larynx inhaler.
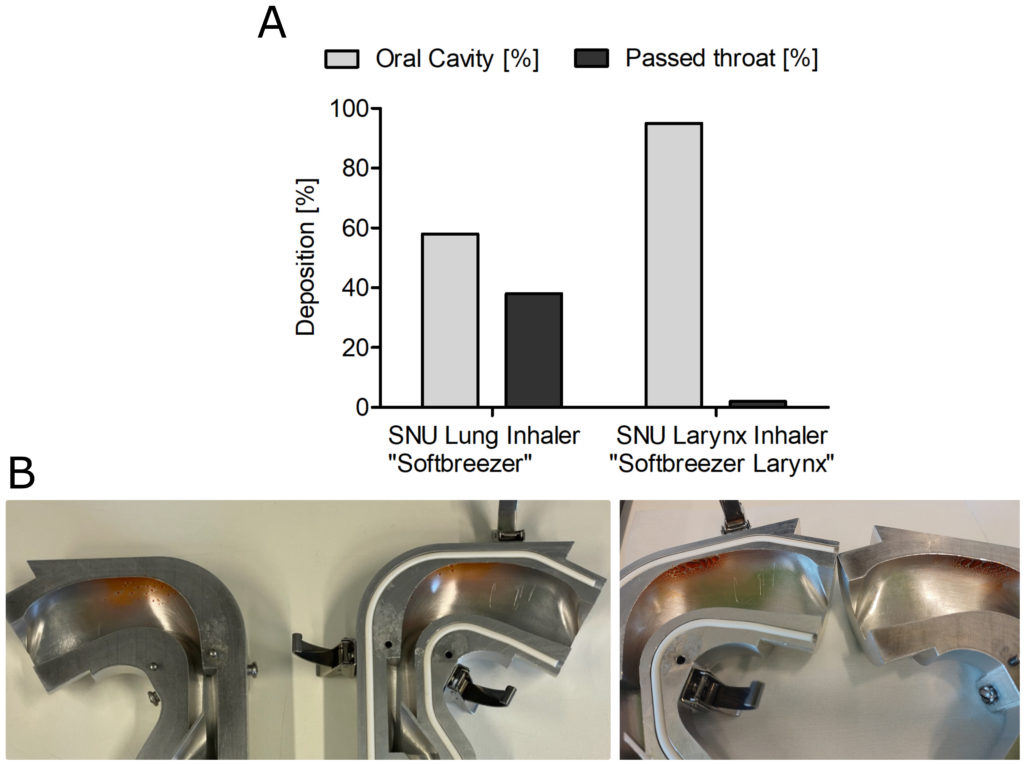
Figure 2: Deposition study of 0.5% fluorescein in 0.9% saline formulation using a next-generation impactor was performed with an airflow rate of 20 L/min. (A) Deposition based on emitted mass; (B) Deposition in an Alberta Idealized Throat (left: lung inhaler; right: larynx inhaler).
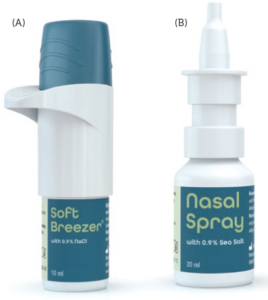
Figure 3: A: The SoftBreezer and the SoftBreezer Larynx are designed in line with a standard portable-inhaler design. They are propellant free and preservative free and generate a fine nebulisation. Exact dosages are generated and the actuation time dependent on the pore size is 1–3 s. B: The nasal spray for the soft mist generation embeds the spray nozzle unit inside the spray nozzle of a standard metered-dose nasal spray.
Another winning fact is that the novelty of the device is completely embedded in the classical device aesthetics of an inhaler and a nasal spray with approved usability profiles and forms familiar to patients (Figure 3).
ONE DEVICE CLASS – DIVERSE TARGETS
Combining spray nozzle technology with pump-based actuation and the option of preservative-free solutions enables the development of similar devices targeting specific regions of the lower and upper airways. As a consequence, synergies in the development of drug products arise as one formulation can potentially be used for local application in different target sites, which allows efficiency in terms of galenic development, manufacturing and stability studies. The targeted local application also generates the potential of optimising drug dose towards a reduced total drug uptake. Moreover, the similar product concepts may allow the patient to use just one user-friendly application technology for drugs in the entire airway, potentially minimising use errors.
REFERENCES
- Newman SP, “Drug delivery to the lungs: challenges and opportunities”. Ther Deliv, 2017, Vol 8(8), pp 647–661.
- Klein MC, Kraus R, “Nose-to-brain drug administration and a novel approach with an old familiar”. ONdrugDelivery, Issue 131 (Apr 2022), pp 22–24.
- George CE et al, “COVID-19 symptoms are reduced by targeted hydration of the nose, larynx and trachea”. Sci Rep, 2022, Vol 12(1), p 4599.
- Lauc G et al, “Fighting COVID-19 with water”. J Glob Health, 2020, Vol 10(1), p 010344.
- de Kruijf W, Ehrhardt C, “Inhalation delivery of complex drugs-the next steps”. Curr Opin Pharmacol, 2017, Vol 36, p 52–57.
- van Geffen GJ et al, “Comparative in-vitro Study of the Trachospray, a New Device for Topical Anaesthesia of the Upper Airway”. Med Devices (Auckl), 2021, Vol 14, pp 9–14.
- Hochrainer D et al, “Comparison of the aerosol velocity and spray duration of Respimat Soft Mist inhaler and pressurized metered dose inhalers”. J Aerosol Med, 2005, Vol 18(3), pp 273–82.
- Newman SP, “Principles of metered-dose inhaler design”. Respir Care, 2005, Vol 50(9), pp 1177–1190.
- Sorino C et al, “Inhalation therapy devices for the treatment of obstructive lung diseases: the history of inhalers towards the ideal inhaler”. Eur J Intern Med, 2020, Vol 75, pp 15–18.
- Lavorini F et al, “What to consider before prescribing inhaled medications: a pragmatic approach for evaluating the current inhaler landscape”. Ther Adv Respir Dis, 2019, Vol 13, p 1753466619884532.
- Work group of the French Society of Otorhinolaryngology, “Consensus document for prescription of nebulization in rhinology”. Eur Ann Otorhinolaryngol Head Neck Dis, 2014, Vol 131(6), p 371–374.
- de Boer AH et al, “In vitro performance testing of the novel Medspray wet aerosol inhaler based on the principle of Rayleigh break-up”. Pharm Res, 2008, Vol 25(5), pp 1186–1192.
- D’Angelo D et al, “Fluorescence-enabled evaluation of nasal tract deposition and coverage of pharmaceutical formulations in a silicone nasal cast using an innovative spray device”. J Adv Res, 2023, Vol 44, pp 227–232.