Citation: Rollings N, Barker C, Vellacott R, “Early Insight: A Flexible, Modular Platform to Disrupt the Blockbuster Manufacturing Model”. ONdrugDelivery Magazine, Issue 103 (Dec 2019), pp 48-52.
Nick Rollings, Colin Barker and Richard Vellacott discuss the drug delivery scenario in the advanced therapy (AT) era. They look at how the current slow-moving blockbuster treatment model will make way for a fast-moving, patient-focused era of personalised medicine – creating new opportunities for flexible, decentralised AT manufacture closer to the patient.
“Digitisation is enabling the emergence of an exciting, dynamic new model underpinned by extensive use of data science and the application of digital software.”
Drug delivery is currently predominantly based on the “blockbuster” model, with a single therapy developed to treat a large patient population. Blockbuster therapies are manufactured in centralised biomanufacturing plants configured to produce large quantities of a single therapy in each batch. But although the blockbuster model has worked for decades, it is now facing disruption from multiple developments occurring in the pharma and broader life sciences sector. The life sciences sector is in the early stages of a “digital” revolution, with potential to significantly disrupt pharma.
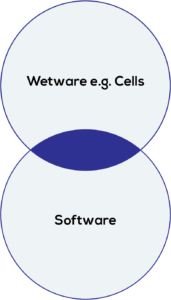
Figure 1: Emerging digital model for life sciences.
Digitisation is enabling the emergence of an exciting, dynamic new model underpinned by extensive use of data science and the application of digital software. Increasingly, artificial intelligence (AI) and machine learning (ML) are also being applied to the life sciences tool chain – influencing all aspects from experimental design through to experimental data capture and analysis (Figure 1).
Despite these developments, pharma and the life sciences sectors still lag behind other industries – and large tech companies see opportunities to enter the life sciences sector, as evidenced by the ambitious recent announcement of the Novartis and Microsoft collaboration to transform medicine with AI.1
NEW THERAPIES
The use of digital tools and large data sets has enabled a transition towards more complex science which, in turn, has allowed a focus on areas of unmet medical need, such as treatments for smaller patient populations. New ATs such as two Novartis gene therapies, Kymriah (tisagenlecleucel) for the treatment of B-cell acute lymphoblastic leukemia, and Zolgensma (onasemnogene abeparvovec) for the treatment of spinal muscular atrophy in children under two years old, are early examples of the increasingly powerful ability to target very specific conditions and smaller patient populations. Currently, however, these therapies have very high cost of goods (CoGs) which has limited the ability of patients to access them.
As the ability to develop therapies for smaller and more targeted patient populations grows, the next decade will see greater stratification of patient populations and increasing personalisation, with a greater variety of cell therapies and gene therapies being developed.
AWAY FROM THE BLOCKBUSTER MODEL
These exciting developments will significantly disrupt the blockbuster model, with current industry tools and approaches being wholly incompatible with cost-effective development and production of bespoke products.
The next decade will require a transition of the “10-year, US$1 billion (£800 million)” development cycle to much leaner development, whilst simultaneously managing high-complexity science and associated high-complexity biological workflows.
It is, however, the manufacture of personalised medicines that will undergo the most significant disruption. Manufacture of high-value therapies will need to be in closer proximity to patients, with a requirement for on-demand manufacturing to suit different patients in varying states of health. There will be a need to accommodate high-complexity biological workflows that can differ considerably between each therapy, in addition to requiring real-time, in-process quality control (QC) analytics, as each therapy becomes a manufacturing batch in its own right.
This departure from the existing reliance upon economies of scale is a significant challenge for slow-moving incumbents in the pharma sector – and a significant opportunity for new entrants that can grasp opportunities in the fast-moving, patient-focused era of personalised medicine.
FUTURE DRUG DELIVERY SCENARIO
The future drug delivery scenario requires a targeted therapy to be manufactured specifically for each patient. This will result in fewer therapy administration occurrences. Following administration, there is then a reliance on frequent diagnostic monitoring and collection of a larger underlying data set to establish the patient’s response to that therapy. Patient response may then trigger the manufacture of a second one-off therapy, modified to suit the patient response. Data and the use of digital tools will be the underpinning enablers of this new personalised medicine era.
This drug delivery scenario places considerable emphasis on the manufacturing of the AT, driving an urgent need to consider alternatives to the current model of central manufacturing reliant on economies of scale. There is a considerable need for new, disruptive manufacturing solutions to deliver the future of personalised medicine.
ALTERNATIVE THERAPY MANUFACTURING MODELS AND CHALLENGES
Decentralised manufacturing is an exciting new opportunity that requires brand new tools and technologies, and which has significant implications for existing pharma models.
The decentralised manufacturing model is seen as a solution to enhance the availability of cell and gene therapies whilst reducing CoGs of the manufactured therapy2 and simultaneously addressing product stability challenges. Autologous therapies often have shelf-lives of only a few hours, necessitating production close to the clinical setting.3
The primary requirements of a decentralised manufacturing capability are enclosed, automated, controlled processes delivering a consistent quality target product profile, at a cost that is affordable to health providers.4
However, by its very nature, decentralised manufacturing closer to the clinic creates secondary challenges, such as integration of the decentralised manufacturing process into existing treatment pathways and minimising the need for extensive retraining of clinical staff. These primary and secondary challenges will need to be addressed by hardware solutions capable of satisfying the following requirements:
- Execution of controlled therapy manufacturing processes, delivering a consistent quality target product profile
- Adherence to good manufacturing practice (GMP) conditions whilst maintaining sterility of bioprocessing equipment
- Processing varying ranges of patient raw material whilst still producing the final therapy to a fixed specification
- Reduce the need for specialist manufacturing staff and for clinical staff to become AT manufacturing experts
- Capable of manufacturing several therapies for different patients simultaneously.
There is also a need for digital solutions capable of collecting and aggregating therapy manufacturing data as a critical input to the determination of patient response to a therapy.
Addressing these requirements will require a new capability – essentially a “flexible, sterile, factory-in-a-box” with digital capabilities closer to the patient. This represents an exciting new paradigm for therapy manufacturing.
COMPLICATION
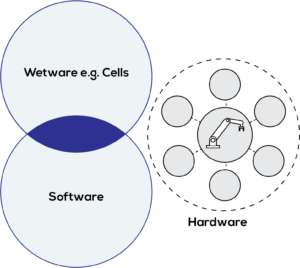
Figure 2: Current laboratory automation scenario.
Evaluation of existing lab automation finds there is still a fundamental gap in the availability of appropriate solutions and technologies to satisfy requirements for decentralised manufacturing of ATs.2
Current lab automation solutions are large, capital-intensive and inflexible, requiring skilled users to operate them. Solutions are orientated towards high-volume production (few tasks, high repetition) rather than low-volume production (multiple tasks, low repetition). Biological workflows are forced to adapt to the fixed functionality of a hardware platform, rather than flexible platforms adapting to the biology.
In addition, the emerging digitisation of biology and fast-paced development of digital tools have resulted in a disconnect between the biology, the software and the actual hardware used to execute experiments. An existing AT manufacturing protocol can require multiple hardware systems from multiple vendors with multiple standards, lacking a common approach – resulting in data silos and an inability to leverage the true potential of emerging AI/ML approaches (Figure 2).
The separation between existing hardware and emerging digital approaches is now constraining personalised medicine manufacturing, where there is a need significantly to reduce CoGs to enable democratisation of personalised therapies.
LESSONS FROM PREVIOUS REVOLUTIONS
Given the fundamental shortcomings of existing lab automation, it is useful to consider previous technology revolutions where a revolution in hardware has previously occurred.
The current generation of lab automation – whilst smaller and less expensive than centralised manufacturing plants – is still expensive, with varying architectures and operating systems, and little standardisation. These systems are comparable to mini computers of the late 1960s that still required dedicated facilities and trained staff.
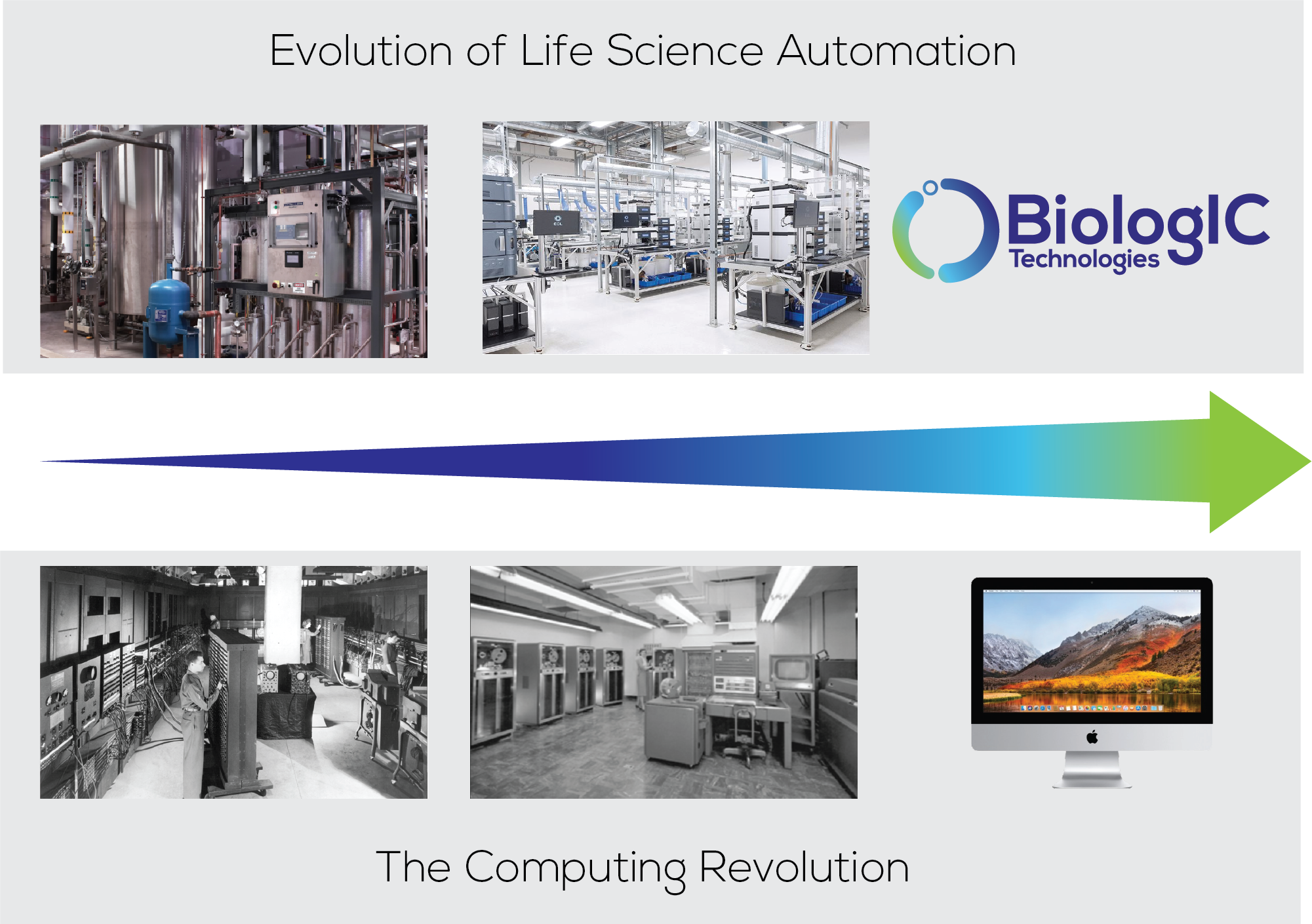
Figure 3: Evolution of life sciences automation and the computing revolution.
It was the development of the desktop PC in the 1980s that created an accessible, affordable, standard yet flexible platform that made computing accessible for the masses (Figure 3). The decentralised manufacture of ATs will require hardware analogous to the desktop PC – a physically small, closed, standard yet flexible automation solution capable of customisation to specific therapy manufacturing workflows.
NEXT-GENERATION MANUFACTURING PLATFORM
BiologIC Technologies is developing a new, disruptive therapy manufacturing platform. It is a standard, yet flexible, platform capable of customisation to suit differing therapies and address the needs of decentralised AT manufacture.
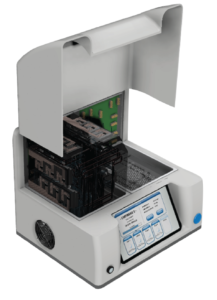
Figure 4: BiologIC’s advanced therapy manufacturing hardware.
The platform represents a disruptive approach to life sciences automation as an affordable and accessible platform. The ability to develop and execute high-value biological workflows rapidly in affordable, application-specific consumables enables life sciences innovation in the same way that the PC made computing accessible for the masses (Figure 4).
HARDWARE ENABLERS
The BiologIC platform exploits know-how from the semiconductor sector to create integrated systems that are not only physically small but also highly powerful. The small yet powerful approach enables miniaturisation of a large factory into a flexible, sterile, factory-in-a-box.
The integrated and miniaturised hardware architecture is particularly advantageous. Integration of several solutions provides an economic approach to the creation of larger systems within a closed automation platform that has a high density of function per volume and low unit cost.
The BiologIC platform enables modular solutions within a standardised hardware architecture. The standard, yet flexible, modular architecture presents an opportunity for risk-sharing schemes across the cell and gene therapy sector.3
Proven, verified modules available to multiple users reduce technical risk and enable the pooling of learning across the AT sector. Rapid reconfiguration of modules to suit specific needs and create custom solutions reduces development cost and risk. In addition to risk mitigation, there are also benefits from the use of custom, modular solutions – such as enhanced protection of manufacturing-related intellectual property. In addition, pooled solutions reduce barriers to entry for smaller therapy developers otherwise unable to afford the creation of automated manufacturing solutions.
This modular approach to complex systems has been extremely successful in the semiconductor sector with the likes of ARM (Cambridge, UK) providing a wide range of microprocessors, modules and supporting tools, enabling customers to create a custom solution for their own needs.
COMPLEMENTARY DIGITAL SOLUTIONS
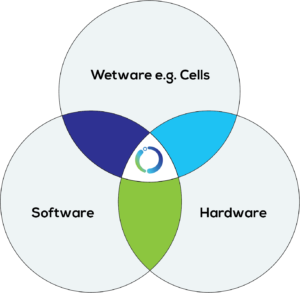
Figure 5: BiologIC Technologies’ platform.
In addition to standardised hardware, the BiologIC platform enables the collection of in-process AT manufacturing data in a standard format. The close integration of hardware and related digital tools allows the adaption of the hardware around the biological workflow and required data – streamlining traditional bioproduct and biotherapy development processes (Figure 5).
BiologIC’s combination of digital biology and digital hardware will enable the rapid creation of lab automation capable of customisation on a project-specific and ultimately experiment-specific basis.
The underlying digital tools used to create the hardware will also enable creation of an ecosystem where workflows/modules/consumables can be shared or created as required in a manner analogous to an Apple or Android app store. The application of ML and AI also represent opportunities to drive value from data collected by the BiologIC hardware during therapy manufacturing.
LONGER-TERM OUTLOOK
Over time, BiologIC sees the opportunity to standardise the hardware used for life sciences and the opportunity to become the supplier of the underlying core technology powering the future of life sciences in a manner analogous to Intel and ARM in the smart devices sector.
CONCLUSION
The AT era will be a fast-moving, patient-focused era of personalised medicine – creating a requirement for new flexible, modular tools and technologies. It is only through the development of these new disruptive solutions that the benefits of AI will be realised.
REFERENCES
- “Novartis and Microsoft announce collaboration to transform medicine with artificial intelligence”. Microsoft News Center, October 2019.
- “Cell Therapy Process Automation, Scalability and Closed Systems”. KNect365, November 2018.
- Mount N et al, “Cell-based therapy technology classifications and translational challenges”. Philos Trans R Soc Lond B Biol Sci, 2015, Vol 370(1680).
- Phacilitate Automation SIG: 2018 Report.