Citation: Fuensalida Pantig GR, “Addressing Complex Biologics with Rational Drug Device Design”. ONdrugDelivery Magazine, Issue 105 (Feb 2020), pp 8-12.
Gene Rhode Fuensalida Pantig reviews drug development over the years, the discovery of biologics as therapeutic agents and the emergence of autoinjectors. In correspondence, Hans Lin, PhD, a Research Fellow from Academia Sinica, Taiwan – who has interests in protein biology and drug discovery – discusses the inherent properties of biologics that should be of prime consideration to pharma companies and drug delivery device developers.
The many advances in molecular biology and biotechnology – from the advent of recombinant DNA technology to the use of panomics1 – have significantly furthered our understanding of the human body’s molecular landscape. At present, scientists and clinicians steer the direction to deliver not just targeted but also truly personalised medicine.
This targeted approach in medicine has come a long way. From the classic antibiotics and antineoplastics, non-selective cytotoxic drug classes that often involve adverse effects for patients, we now shift to biologics – therapeutic molecules which try to correct biochemical pathways or inhibit aberrant cells or proteins. Alongside the development of biologics is the need to develop novel delivery systems that not only consider the molecule but also the end receivers of drug therapy – the patients. As well as the standard prefilled syringes for the assisted administration of biologics, recently developed prefilled pen and autoinjectors for patient self-administration have also found their space in molecular medicine.2
THE CLASSICAL APPROACH TO DRUG DISCOVERY AND DESIGN
In the past, drug development and discovery have mainly focused on small molecules, primarily owing to their oral bioavailability and their affinity to bind a druggable target. For a compound to be considered a lead in rational drug discovery, a classic requirement would be to satisfy Lipinski’s “rule of five” (Ro5).3
The Ro5 is a set of physicochemical properties that allows the compound to be absorbed in the gastrointestinal system.4,5 In general, the rule outlines that a lead compound should be relatively small in molecular size and, owing to its chemical properties, lipophilic in nature. While the rule has always been considered to determine the fate of whether a drug can even reach its site of action, most compounds that do not satisfy it are left unstudied.
ORAL SMALL-MOLECULE DRUGS AND THE DRUGGABILITY DILEMMA
“Of the many challenges in delivering precision medicine, the drug itself is still the rate-limiting step.”
Druggability,6 on the other hand, refers to the likelihood of a target – oftentimes a protein involved in disease states – being modulated by a drug. Thus, while a potential drug may be considered absorbable, it must then bind to its druggable target atthe site of action. This binding is expected to elicit a cascade of events, eventually alleviating disease conditions – at least at the symptom level.
However, not all disease-specific molecules or proteins are said to be druggable.7 In fact, of the ~21,700 proteins identified in the human proteome by the year 2002, only 3000 are estimated to be druggable.8 Putting this into perspective, subset data published in 2006 states that only 186 human proteins have been targets of all US FDA-approved oral small-molecule drugs of the same year – a number which is less than 10% of the druggable, potentially disease-related proteins.9
With only around 10% of the druggable human proteome addressed, there has been a spark of interest in discovering novel treatment modalities. After all, the remaining ~90% accounts for proteins and protein-protein interactions that are involved in various important biochemical pathways. At such a molecular level, any aberration would almost always result in a very specific disease state.
Simply put, a large portion of the druggable proteome is “undruggable” for oral small-molecule drugs. Taking a fast track into 21st-century therapeutics, biologics – molecules administered in recently developed delivery systems such as prefilled syringes and autoinjectors – have been reigning supreme to address the task.2
OVERCOMING THE RATE-LIMITING STEP IN MEDICINE
Of the many challenges in delivering precision medicine, the drug itself is still the rate-limiting step. In recent years, what remained elusive for small-molecule therapy has been taken over by biomolecules as therapeutics.10 Scientists have started tapping into the potential use of large-molecule biologics.
“With only around 10% of the druggable human proteome addressed, there has been a spark of interest in discovering novel treatment modalities.”
In general, biologics are defined as pharma compounds synthesised or extracted from a “living” or biological source.11 For the purpose of this article, we are focusing on biologics for therapeutic purposes – monoclonal antibodies (MAbs) and recombinant proteins, most of which are the result of harnessing recombinant DNA technology. While the first recombinant biologic was approved for clinical use in the 1980s, it was not until the dawn of the 21st century that we witnessed a steady trajectory in the research, development and use of these compounds.12
The successful use of biologics in clinical applications has come a long way. Since the 1982 FDA approval of human insulin – the first recombinant biologic – development of biologic compounds has continued to be met with failures and triumphs. As early as 2005, researchers predicted the shift from organic chemistry to protein biology in medicine.13 Since the concept of druggability has been discussed earlier, it is important to put on record that the field recognises that protein biologics are required to modulate and disrupt disease-related proteins and protein-protein interactions, which are often characterised with low druggability.14 You could say the undruggable then becomes druggable through biologics.
With the current approach, caveats in druggability are circumvented by using biologics to modulate disease-specific proteins and their pathways.7 From a symptom-based alleviation of the disease, now the molecular underpinnings of diseases are addressed, with recent biologics indicated for cancer, inflammation-related conditions, diabetes and migraine, to name but a few.
Biological medicines, especially proteins and antibodies, hold various advantages over small-molecule therapies. Proteins are highly specific in nature and function, and thus their action towards biological processes are precise and do not cause adverse effects.12 The same is true for antibodies – versatile molecules that play a huge role in targeted therapy of diseases.12,15 Biologic drugs, however, don’t come without limitations and challenges.
UNDERSTANDING THE DELIVERY OF BIOLOGICS
The knowledge of the general physicochemical properties of biologics is of vital importance in translating these drugs into deliverable formats for effective patient administration. Since biological therapeutics are usually highly ordered but complex assemblies of long-chain peptides, they are of significantly high molecular weight and viscous in terms of their chemical and physical nature. In a discussion with SHL on this subject, Dr Hans Lin, a Research Fellow in the Institute of Biological Chemistry at Academia Sinica in Taiwan, communicates the inherent properties of biologics that should be of prime consideration to pharma companies and drug delivery device developers.
A researcher in the field of protein biology and drug discovery, Dr Lin points out that knowledge of the general pharmacokinetic properties of biologics is important. Further, he explains that it is during the preclinical and clinical studies when the biological drug’s potency and effective dose, dosing frequency requirements and effective administration route is identified. With biological drugs varying in dosing and dosing frequency requirements, foresight is thus imperative for the success of a combination drug product strategy that is not only effective but acceptable for the patient.2,13,15,16
For example, clinical studies on the first systemic therapy for atopic dermatitis – an mAb – indicated the need for a higher concentration of the drug to achieve pharmacological efficacy. In terms of formulation, this translates to a biological drug that would require a higher volume in solution. The delivery format, on the other hand, would then require one that could be administered by patients without the need for intervention by a healthcare professional and with less drug administration frequency.
Case in point, a 2.25 mL autoinjector based on the Molly® platform could be one of the first to be approved by regulatory agencies as part of a combination product for the treatment of atopic dermatitis, asthma and a few other indications.17 Molly® is an example of a single-use autoinjector that can accommodate complex biologics in the 0.1-2.25 mL range. For many years, 1.0 mL was considered the maximum volume for a subcutaneous injection, a measure adjusted to 1.5 mL by Mathaes and colleagues. Now, we are starting to see the first drugs in the 2 mL volume range being provided to patients in autoinjectors for self-treatment.17,18
The case for Molly® 2.25 as a combination product comes as a precedent in the evolving self-injection delivery of complex biologics for therapy. Along with the increasing development of biologics, it is of equal interest to note that this is one of the first autoinjectors for self-injection in the higher volume range – a precedent to consider for future development of drugs for self-injection.
COMBINATION PRODUCTS ON A FORMULATION PERSPECTIVE
From a formulation perspective, it is important for researchers and drug device design engineers to understand the characteristic nature and properties of biologic drugs. The bioavailability of these large molecules – or the amount of the drug which reaches the body’s systemic circulation to elicit an effect – is absolutely compromised in the oral state. Biologics require carefully designed medical devices to ensure proper parenteral drug administration and absorption into the body’s systemic circulation. As such, this understanding is a crucial cause for the recent development of biologics in autoinjector delivery formats2,16 by pharma companies and drug device developers, wherein acceptance of the format by patients is increasing.27
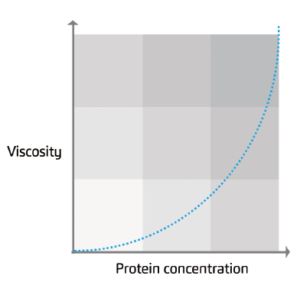
Figure 1: Generalised graph of the relationship between protein concentration and viscosity.
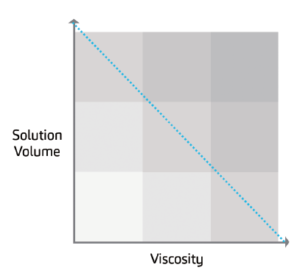
Figure 2: Generalised graph of the inverse relationship between protein viscosity and solution volume.
Complex biologics, like monoclonal antibodies (mAbs), typically require higher concentrations to produce clinically relevant effects. For such molecules, viscosity exponentially increases as a function of protein concentration (Figure 1).18 Therefore, for a combination product to succeed in therapy, one must delineate drug device design in relation to the properties of the drug while considering patient-related factors in terms of drug administration.
In the medical device field, the Bertha® autoinjector is an example of a device that has been designed to address higher viscosity drug preparations. High protein concentrations that are characterised by viscosities of up to 60 cP can be delivered by the device, and its two-step operation is indicative of simplicity for patient self-injection.
“From a patient standpoint, the practical aspects surrounding a combination product are critical, with usability as the prime focal point.”
On the other hand, concentrated protein biologics may require specific excipients to prevent aggregation and the addition of an optimal volume of diluent to increase drug stability. The increase in dose volume, in turn, may positively affect protein stability and lower viscosity. This could be illustrated in the inverse proportionality of protein viscosity and volume of the solution
(Figure 2).18 For biologics that may require volumes beyond 2 mL, cartridge-based devices may prove to be helpful for successful drug delivery. The Maggie® autoinjector, for example, uses a standard 3 mL cartridge.
On the basis of the intrinsic nature of protein biologics, the need for delivery devices that can accommodate higher fill volumes of biologics is implicit (Figure 3). In theory, biologics such as mAbs require higher quantities to reach the therapeutic dose.18 On account of its concentration, this means that such biologics should be packed in a volume acceptable for patient administration but interspersed enough to prevent physicochemical challenges.
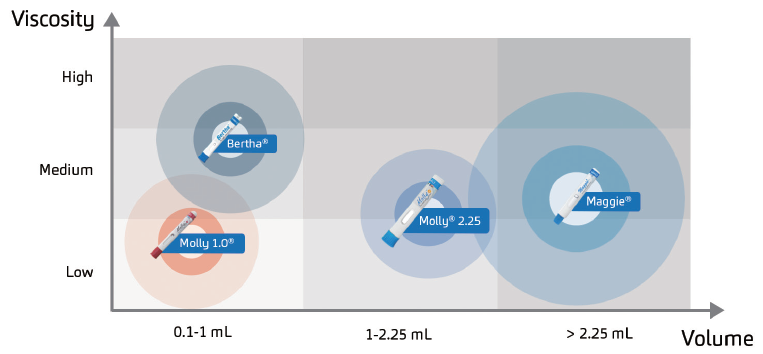
Figure 3: Examples of currently available drug delivery modalities for complex biologics.
As was exemplified earlier by the case for Molly® 2.25, explorations of therapeutic volumes for biologics beyond 2 mL are underway. With the rise in the development of bigger and more complex protein biologics, delivery devices addressing these biologics and optimised for self-treatment – like Maggie® – are crucial.
CURRENT AND FUTURE DIRECTIONS FOR COMBINATION PRODUCTS
With the current field of medicine approaching a more personalised way of treating disease conditions, interest in the development of biologics will remain. Since the core of precision medicine – which is to understand and address the underlying cause of diseases in individual patients19 – is addressed by biological treatments, the research to discover biologics, biobetters and biosimilars will continue.20 With patients as the end receivers of these treatment modalities, the continued success of biologics as combination products will depend on even tighter collaborative efforts between the researchers and developers involved.
From a patient standpoint, the practical aspects surrounding a combination product are critical, with usability as the prime focal point. In retrospect, some of the usability challenges related to small-molecule, oral treatments included difficulty opening tablet containers and blister packs. While these challenges were identified through research, there are few published papers about this aspect of medicine.21,22
For biologics, now finding their space in targeted medicine, their success as combination products will depend on even tighter collaborative efforts between the researchers and developers involved. This means a well-thought-out and concerted development of the combination product – from research and formulation, primary packaging and secondary packaging through to patient usability, where the final product ideally would have an optimal balance between viscosity, volume and injection frequency. Thus, in translating these biological molecules that have varying intrinsic properties such as potency, viscosity and volume into a deliverable format, the need for their co-development with autoinjectors that complement these varying requirements will continue.
CONCLUSIONS
The field of therapeutics has certainly progressed through discoveries in molecular biology and biotechnology. These discoveries have made the development and production of complex biologics possible, and interest in biological therapeutics is strong (Figure 4).10,23,24
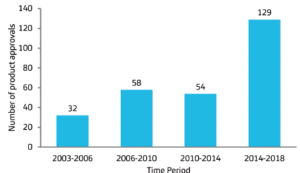
Figure 4: Regulatory approval of biopharmaceuticals over the years. The numbers pertain to the US and European Union regulatory approvals of recombinant biologics over the survey period.
Along with rational drug design – a longstanding concept in pharmacological science – the success of a combination product for effective patient therapy relies on the rational design of drug devices. The significance of autoinjectors as drug delivery modalities for complex biologics will continue, as it delineates drug device design with the intrinsic properties of drugs and drug administration by patient self-injection. With the advent of digital technologies, it is also of interest that adaptation of medical software and digital implementations for self-injection devices are underway.25,26
Historically, medical devices were not perceived by the healthcare field with the same significance as that of the drug itself, as evidenced by the previous regulatory stipulations for devices.27 Now, it could be said that therapeutics in the era of biologics require drug delivery devices as indispensable patient tools. Firstly, these drug devices serve to administer complex drug formulations targeted for specific patients. Secondly, with the advent of connected therapeutics – these devices serve to connect the patient to healthcare professionals and patient support programs in order to optimise therapeutic outcomes.25,26
Further development in drug delivery devices and combination products will truly allow the generation of populated patient data to inform pharma companies, drug-device developers, regulatory agencies and the patients about the absolute value of the drug.
For combination products, the interplay of the drug delivery device in relation to the drug’s pharmacokinetics is evident, as the drug device plays a role in the fine tuning of drug formulation, dosage, doseadministration and periodicity of injection. As patients are the end receiver of these combination therapeutics, evaluation of patient acceptance is of importance. Recently, an in-depth review has positively reported on patient compliance with self-injection devices such as autoinjectors.28 Finally, as researchers discover novel biological compounds, the need for injection devices addressing the varying viscosities and formulation volumes will continue.
Our correspondent in this article, Hans Chun-Hung Lin is a Research Fellow at the Institute of Biological Chemistry, Academia Sinica – Taiwan’s national research academy. He has a PhD in Chemistry from the Scripps Research Institute (CA, US), and from 1995 to 1997 pursued his postdoctoral fellowship at Harvard Medical School (MA, US). Dr Lin’s multidisciplinary research interests include protein biology, particularly enzymology and glycobiology, as well as drug discovery. At present, he serves as the Director of Academic Affairs at Academia Sinica.
REFERENCES
- Sandhu C, Qureshi A, Emili A, “Panomics for Precision Medicine”. Trends Mol Med, 2017, Vol 24(1), pp 85–101.
- Gurman P et al, “Prefilled devices for parenteral applications”. Expert Rev Med Devices, 2014, Vol 11(2), pp 205–223.
- Fauman E, Rai B, Huang E, “Structure-based druggability assessment — identifying suitable targets for small molecule therapeutics”. Curr Opin Chem Biol, 2011, Vol 15(4), pp 463–468.
- Veber D et al, “Molecular Properties That Influence the Oral Bioavailability of Drug Candidates”. J Med Chem, 2002, Vol 45(12), pp 2615–2623.
- Le J, “Drug Absorption”. MSD Manual (Professional Version), May 2019.
- Owens J, “Determining druggability”. Nature Rev Drug Discov, 2007, Vol 6(3), pp 187–187.
- Mabonga L, Kappo A, “Proteinprotein interaction modulators: advances, successes and remaining challenges”. Biophysical Reviews, 2019, Vol 11(4), pp 559–581.
- Hopkins A, Groom C, “The druggable genome”. Nature Rev Drug Discov, 2002, Vol 1(9), pp 727–730.
- Overington J, Al-Lazikani B, Hopkins, A, “How many drug targets are there? Nature Rev Drug Discov, 2006, Vol 5(12), pp 993–996.
- Walsh G, “Biopharmaceutical benchmarks 2018”. Nature Biotechnol, 2018, Vol 36(12), pp 1136–1145.
- Chan J, Chan A, “Biologics and biosimilars: what, why and how?”. ESMO Open, 2017, Vol 2(1).
- Leader B, Baca Q, Golan D, “Protein therapeutics: a summary and pharmacological classification”. Nature Rev Drug Discov, 2008, Vol 7(1), pp 21–39.
- Frokjaer S, Otzen D, “Protein drug stability: a formulation challenge”. Nature Rev Drug Discov, 2005, Vol 4(4), pp 298–306.
- Modell A, Blosser S, Arora P, “Systematic Targeting of Protein– Protein Interactions”. Trends Pharmacol Sci, 2016, Vol 37(8), pp 702–713.
- Brekke O, Sandlie I, “Therapeutic antibodies for human diseases at the dawn of the twenty-first century”. Nature Rev Drug Discov, 2003, Vol 2(1), 52–62.
- Jain D, Mahammad S, Singh P, Kodipyaka R, “A Review on Parenteral Delivery of Peptides and Proteins”. Drug Dev Ind Pharm, 2019, Vol 1–33.
- Bantz S, Zhu Z, Zheng T, “The atopic march: progression from atopic dermatitis to allergic rhinitis and asthma”. Allergy, Asthma Immunol Res, 2011, Vol 3(2), p 67.
- Mathaes R, Koulov A, Joerg S, Mahler H, “Subcutaneous Injection Volume of Biopharmaceuticals— Pushing the Boundaries”. J Pharm Sci, 2016, Vol 105(8), pp 2255–2259.
- Dugger S, Platt A, Goldstein D, “Drug development in the era of precision medicine”. Nature Revs Drug Discov, 2017, Vol 17(3), pp 183–196.
- Kesik-Brodacka M, “Progress in biopharmaceutical development”. Biotechnol Appl Biochem, 2017, Vol 65(3), pp 306–322.
- Van Geffen E, Meuwese E, Philbert D, Bouvy M, “Problems with Medicine Packages: Experiences Reported to a Dutch Medicine Reporting System”. Ann Pharmacother, 2010, Vol 44(6), pp 1104–1109.
- Beckman A, Bernsten C, Parker M, Thorslund M, Fastbom J, “The Difficulty of Opening Medicine Containers in Old Age: A Population- Based Study”. Pharm World Sci, 2005, Vol 27(5), pp 393–398.
- Walsh G, “Biopharmaceutical benchmarks 2010”. Nature Biotechnol, 2010, Vol 28(9), pp 917–924.
- Walsh G, “Biopharmaceutical benchmarks 2014”. Nature Biotechnol, 2014, Vol 32(10), pp 992–1000.
- Makin S, “The emerging world of digital therapeutics”. Nature, 2019, Vol 573(7775), pp S106-S109
- Gordon W, Stern, A, “Challenges and opportunities in software-driven medical devices”. Nature Biomed Eng, 2019, Vol 3(7), pp 493–497.
- Merrill R, “Regulation of Drugs and Devices: An Evolution”. Health Affairs, 1994, Vol 13(3), pp 47-69.
- Maniadakis N et al, “A Targeted Literature Review Examining Biologic Therapy Compliance and Persistence in Chronic Inflammatory Disease to Identify the Associated Unmet Needs, Driving Factors, and Consequences”. Adv Ther, 2018, Vol 35(9), pp 1333-1355.