To Issue 158
Citation: Miller E, Jameson H, “Bubble-Wrapped Remedies: How Lipid-Encased Therapeutics Could Transform Inhalation”. ONdrugDelivery, Issue 158 (Apr 2024), pp 20–23.
Ethan Miller and Heather Jameson shed light on some of the intricacies that lipid-encapsulated therapeutics bring to the realm of inhalation therapies, and some considerations on how the type of inhalation device selected may help to mitigate issues around formulation stability and efficient delivery to target cells.
In the post-covid-19 era we have witnessed a notable shift in vaccine development with the advent of lipid nanoparticle (LNP) vaccines, representing a ground breaking approach to immunisation, opening new avenues for developing vaccines against various pathogens and reshaping the future of preventive medicine. LNPs offer a means of “bubble-wrapping” therapeutic cargo, protecting it from degradation and facilitating its internalisation into cells. Additionally, RNA-carrying LNPs provide a unique opportunity to treat respiratory diseases resulting from inherited anomalies, using techniques such as gene editing, silencing or replacement therapies to treat the root cause of the diseases.1
The systemic delivery of LNP therapies via intravenous (IV) injection has significant challenges for pulmonary disorders; the first being the coating of LNPs with apolipoprotein E (ApoE) protein in blood plasma. This ApoE coating causes LNPs to be preferentially delivered to the liver rather than desired pulmonary tissues. Such challenges in IV injection of pulmonary therapies make inhalation a promising avenue for LNP delivery.
However, the delivery of lipid-encapsulated therapies via inhalation is not without its own challenges, with mucus2 and lung surfactant1 impacting the efficacy of LNP therapeutic delivery to target cells. Further challenges are presented by the mechanical or thermal stresses exerted on LNPs during the aerosolisation or drying processes. This article will explore some of the recent developments that are aiming to overcome these challenges, and unlock the potential of inhaled LNP therapeutics.
NANO CARGO SHIPS: WHAT IS LNP ENCAPSULATION?
LNPs are tiny particles typically ranging from 10 to 200 nm in diameter. These nanoparticles can be designed to target specific tissues or cells, release their cargo in response to specific triggers (such as pH or enzymes), and improve the bioavailability and efficacy of therapeutic compounds.
LNPs can be used to encapsulate therapeutic compounds, such as drugs or genetic material (like RNA or DNA). The LNP provides a protective shell for nucleic acids and other therapeutic cargos,as illustrated in Figure 1, preventing enzymatic degradation until the cargo is successfully delivered to the target cells.3 The successful delivery of the nucleic acid cargo into the target cell is known as “transfection”.
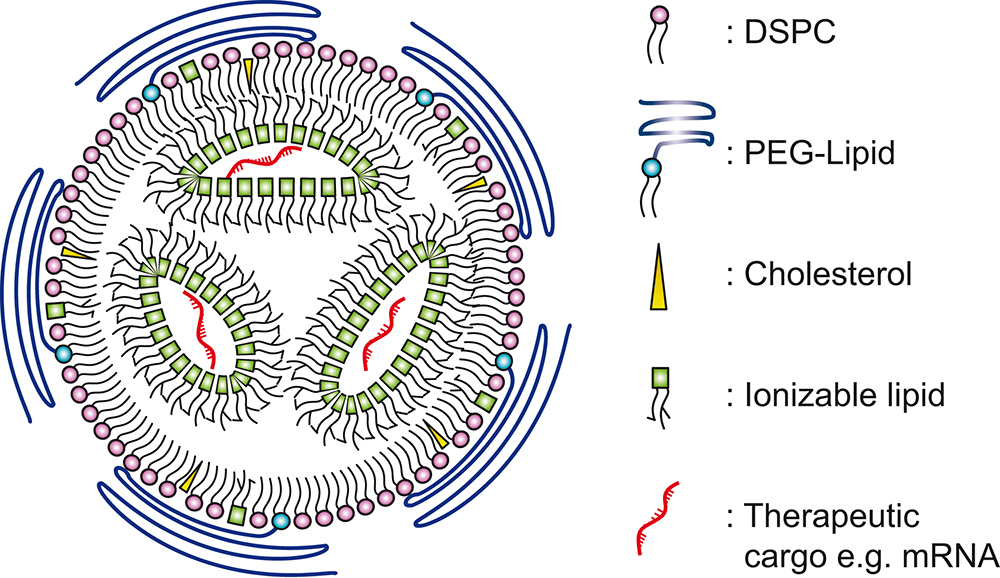
Figure 1: Simplified illustration of LNP and its constituent components. Based on illustrations from Albertsen (2022).3
A wide variety of therapeutics can be delivered via LNP encapsulation, including small molecule drugs, nucleic acids (such as DNA, RNA, siRNA), vaccines, proteins, peptides, imaging agents and gene editing tools. The approach has applications not only for drug delivery and gene therapy but also in diagnostics and imaging. LNP encapsulation can enable the development of more effective and targeted therapies for various diseases and conditions, offering potential improvements in efficacy, safety and patient outcomes.
“To mitigate the impact of shear forces on LNP rupture and loss of efficacy, extensive research is underway into shear-reducing aerosolisation techniques.”
SHEAR BRILLIANCE: MITIGATING THE IMPACT OF SHEAR STRESS ON LNPS
Nebulisers and soft-mist inhalers aerosolise liquid formulations, generating a fine mist that the patient inhales to achieve direct delivery of therapeutics to the respiratory tract. The aerosolisation process may subject the LNPs to high shear forces, especially during nebulisation or when passing through narrow orifices. These forces can cause structural alterations or complete rupture of the lipid bilayer, leading to the premature release of encapsulated agents and, consequently, a significant loss of efficacy.4
Nebulisation Mechanisms
To mitigate the impact of shear forces on LNP rupture and loss of efficacy, extensive research is underway into shear-reducing aerosolisation techniques. Vibrating mesh nebulisers (Figure 2A) appear to be a more suitable method of gene therapy delivery compared with jet and ultrasonic nebulisers.4 Conventional jet nebuliser designs (Figure 2B) often require a baffle in the aerosolisation mechanism, creating large shear forces in the aerosolised product as it exits the nozzle. Instead, vibrating mesh nebulisers use a microscopic mesh vibrating at ultrasonic frequencies to create a fine mist from liquid medications. The absence of baffles within the vibrating mesh nebulisers reduces shear stress and damage to the LNPs and their cargo.5 Future vibrating mesh nebuliser technologies will likely incorporate bespoke mesh technologies tailored for specific drugs and therapies, optimising drug delivery for the particular formulation of LNP encapsulated therapy.
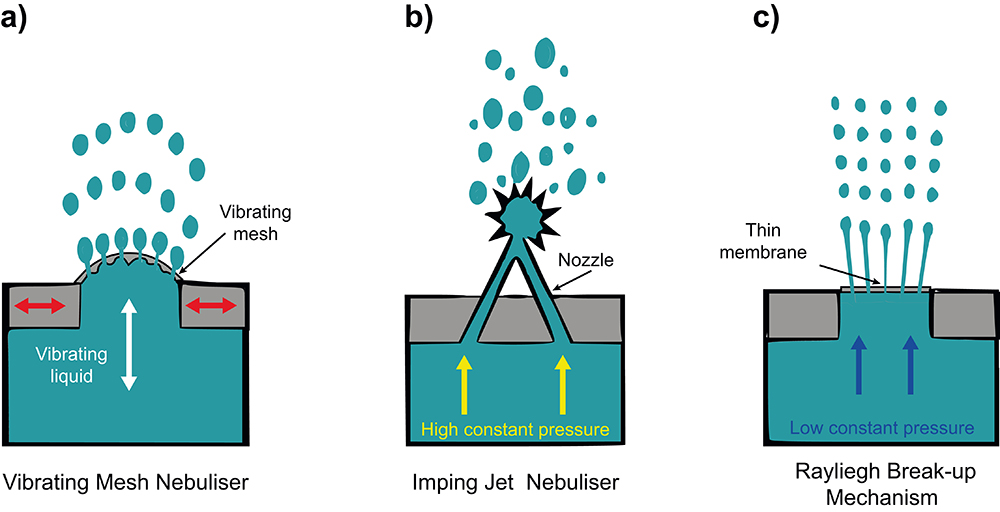
Figure 2: Methods for nebulisation. (A) Vibrating mesh nebuliser; a flexible mesh mounted on an actuator that stretches and vibrates to aerosolise the contained liquid. (B) Imping jet nebuliser: two jets collide at a high velocity, breaking up into small droplets. (C) Rayleigh break-up: fluid is pushed through a thin nanopore membrane at low pressure, creating multiple jets that subsequently break up in equally sized droplets. Based on illustrations from van Rijn et al (2023).6
In addition to the established nebuliser aerosolisation mechanisms, two further mechanisms have been employed in soft-mist inhalers: impinging jets (Spiriva Respimat (Boehringer Ingelheim)) and Rayleigh break-up (Medspray). A study comparing three nebulisation techniques, including vibrating mesh, impinging jets (Figure 2B) and a novel design employing Rayleigh break-up (Figure 2C), found that reducing the energy level required to aerosolise the formula is critical to safeguarding the integrity of the LNP and cargo.6 The Rayleigh break-up mechanism requires much lower energy input to aerosolise the formula (2 J/g verses >20 J/g for other mechanisms), and hence was found to be the most successful. However, the nanoscale pores required may present manufacturing and maintenance challenges.
Lipid Composition Optimisation
The optimisation of lipid composition for increased resilience and developing novel formulations with inherent protective properties against mechanical stress is also a strategy under investigation. A recent study showed optimising LNP composition could improve mRNA transfection after aerosolisation via a mesh nebuliser, by switching the ionisable lipid for a more saturated variant. This resulted in the aerosolised LNP being more effective at transfecting gene therapies to lung tissues in mouse models.7
The promising developments in nebulisation technology and optimisation of lipid composition suggest that the challenges associated with the mechanical stresses of nebulisation are not insurmountable. Hence, we should expect that liquid formulations and their associated inhaler devices will remain relevant in the future development of inhaled LNP drugs.8
“Studies have shown that the optimisation of the excipient, the inactive vehicle of the LNP with the dry powder formulation, can significantly improve formulation characteristics.”
POWDER PIONEERS: LNPS IN DRY POWDER INHALERS
In addition to the challenge of shear forces, formulation in a liquid state increases the tendency of premature protein drug degradation during storage. The alternative to liquid nebulisation is to first formulate as a dry powder and deliver using a dry powder inhaler (DPI). DPIs have the benefit of convenience compared with nebulisers, being generally smaller and more portable, as well as cheaper. Compared with pressurised metered dose inhalers, they do not require propellant, which would need to be compatible with the drug.
Drying Techniques
Spray-drying is a common method employed for producing therapeutics as powders. The lipid solution containing the API is atomised into fine droplets using a nozzle. These droplets are dried rapidly in a heated chamber, resulting in the formation of solid LNPs encapsulating the drug, suitable for pulmonary delivery through DPIs.
Spray-dried LNP formulations have been shown to be able to achieve low residual moisture levels (below 0.5%).
When inhaled, the dry powders form particles sized between 1 and 5 μm, which are ideal for deep inhalation into the lung and deposition in the alveolar region. Importantly, recent studies have shown that spray-dried LNPs retain their capability for transfection and can be used to deliver gene therapies.9 Advanced drying techniques are also being developed, with one study preparing siRNA-encapsulated solid LNPS by thin-film freeze-drying showing promising results.10
“To mitigate the impact of shear forces on LNP rupture and loss of efficacy, extensive research is underway into shear-reducing aerosolisation techniques.”
Excipients
Studies have shown that the optimisation of the excipient, the inactive vehicle of the LNP with the dry powder formulation, can significantly improve formulation characteristics. The combination of both mannitol and leucine has been found to create much smoother and spherical LNPs when observed through a scanning electron microscope, and the addition of ethanol in the inlet feed aided in reducing the particle size of the dry powder product.11
Historically, the choice of excipients approved for inhalation has been limited, with lactose and magnesium stearate being, by far, the most widely used compounds in inhaled drug products.12 Diversification of approved excipient compounds will aid the future development of stable inhaled LNP therapeutics.8
PUFFING PROGRESS: FUTURE CONSIDERATIONS FOR LNP IN INHALATION DEVICES
LNP therapeutics and inhalers are poised to drive innovation in pulmonary drug delivery, enhancing efficacy, safety and the patient experience. As highlighted in this article, one method of advancement lies in the refinement of LNP formulations to optimise their physicochemical properties for targeted lung delivery. This involves tailoring lipid composition, surface modifications and encapsulation strategies to improve stability, bioavailability and cellular uptake within the lungs.
In parallel, advances in nanotechnology, such as the development of functionalised nanoparticles for targeted drug delivery and imaging, hold promise for synergistic approaches to disease diagnosis and treatment. By combining LNP-based therapeutics with imaging agents or nanoparticles, clinicians can gain insights into disease progression, monitor treatment response and tailor therapy for optimal outcomes.
The integration of innovative technologies in the inhalation devices is also promising, for example, the incorporation of microfluidics architectures. A recent study demonstrated a microfluidic system for producing aerosolised nanoparticles for inhaled mRNA therapy.13 The particles were generated by individual microfluidic nozzles for precise droplet ejection, allowing for the low shear generation of droplets with minimal LNP disruption, aggregation or cargo leakage.
Overall, the future of LNP therapeutics and inhalers lies in multidisciplinary collaboration, technological innovation and a patient-centric approach to respiratory care. By harnessing the potential of LNPs and inhalation devices, researchers and clinicians can revolutionise the management of lung diseases, improving quality of life and clinical outcomes for patients worldwide.
REFERENCES
- Kassab G et al, “Inhalable Gene Therapy and the Lung SurfactantProblem”. Nanoletters, 202, Vol 22, pp 10099–10102.
- Kim J et al, “Engineering Lipid Nanoparticles for Enhanced Intracellular Delivery of mRNA through Inhalation”. ACS Nano, 2022, Vol 16(9), pp 14792–14806.
- Albertsen CH et al, “The role of lipid components in lipid nanoparticles for vaccines and gene therapy”. Adv Drug Deliv Rev, 2022, Vol 188, p 114416.
- Arulmuthu E et al, “Studies on Aerosol Delivery of Plasmid DNA Using a Mesh Nebulizer”. Biotechnol Bioeng, 2007, Vol 98(5), pp 939–955.
- McCarthy S, González H, Higgins B, “Future Trends in Nebulized Therapies for Pulmonary Disease”. J Pers Med, 2020, Vol 10(2), p 37.
- Van Rijn CJM et al, “Low energy nebulization preserves integrity of SARS-CoV-2 mRNA vaccines for respiratory delivery”. Sci Rep, 2023, Vol 13(1), p 8851.
- Lewis M et al, “Optimization of ionizable lipids for aerosolizable mRNA lipid nanoparticles”. Bioeng Transl Med, 2023, Vol 8(6), p e10580.
- Leong EWX, Ge R, “Lipid Nanoparticles as Delivery Vehicles for Inhaled Therapeutics”. Biomedicines, 2022, Vol 10(9), p 2179.
- Zimmermann CM et al, “Spray drying siRNA-lipid nanoparticles for dry powder pulmonary delivery”. J Control Release, 2022, Vol 351, pp 137–150.
- Wang J-L et al, “Aerosolizable siRNA-encapsulated solid lipid nanoparticles prepared by thin-film freeze-drying for potential pulmonary delivery”. Int J Pharm, 2021, Vol 596, p 120215.
- Sarode A et al, “Inhalable dry powder product (DPP) of mRNA lipid nanoparticles (LNPs) for pulmonary delivery”. Drug Deliv Transl Res, 2024, Vol 14(2), pp 360–372.
- “Accelerating excipient development for inhalable dosage forms with QbD”. Manufacturing Chemist, May 8, 2021.
- Kim J et al, “Microfluidic platform enables shear-less aerosolization of lipid nanoparticles for messenger RNA inhalation”. 2024, bioRxiv Preprint.
Previous article
HOW TO CHOOSE THE RIGHT INTRANASAL VACCINE DEVICENext article
THE EVOLUTION OF PULMONARY & NASAL DRUG DELIVERY