To Issue 158
Citation: Saldanha S, Santos L, Churro R, “High-Dose Delivery Platform for Crystalline DPIs”. ONdrugDelivery, Issue 158 (Apr 2024), pp 55–59.
Susana Saldanha, Lídia Santos and Rui Churro, discuss the benefits of crystalline formulations for inhalation devices.
“High concentrations of drug at the site of action could enable a reduction in dose and dosing frequency, and, subsequently,
potential side effects.”
Respiratory drug delivery has diversified the pipeline and portfolio of diseases that can be treated through this route, including already approved anti-infective medicines for cystic fibrosis or influenza patients, and others in development for pulmonary arterial hypertension, idiopathic pulmonary fibrosis and other rare lung diseases. Also, the doses and molecules delivered to the lungs have increased in the last years from a few micrograms to milligrams, as seen in Figure 1.1,2 This growth in the delivery of high doses has been observed in pharmaceutical compounds, such as antibiotics, vaccines, proteins and peptides, as well as for the treatment of acute emergency indications via the inhalation route.3
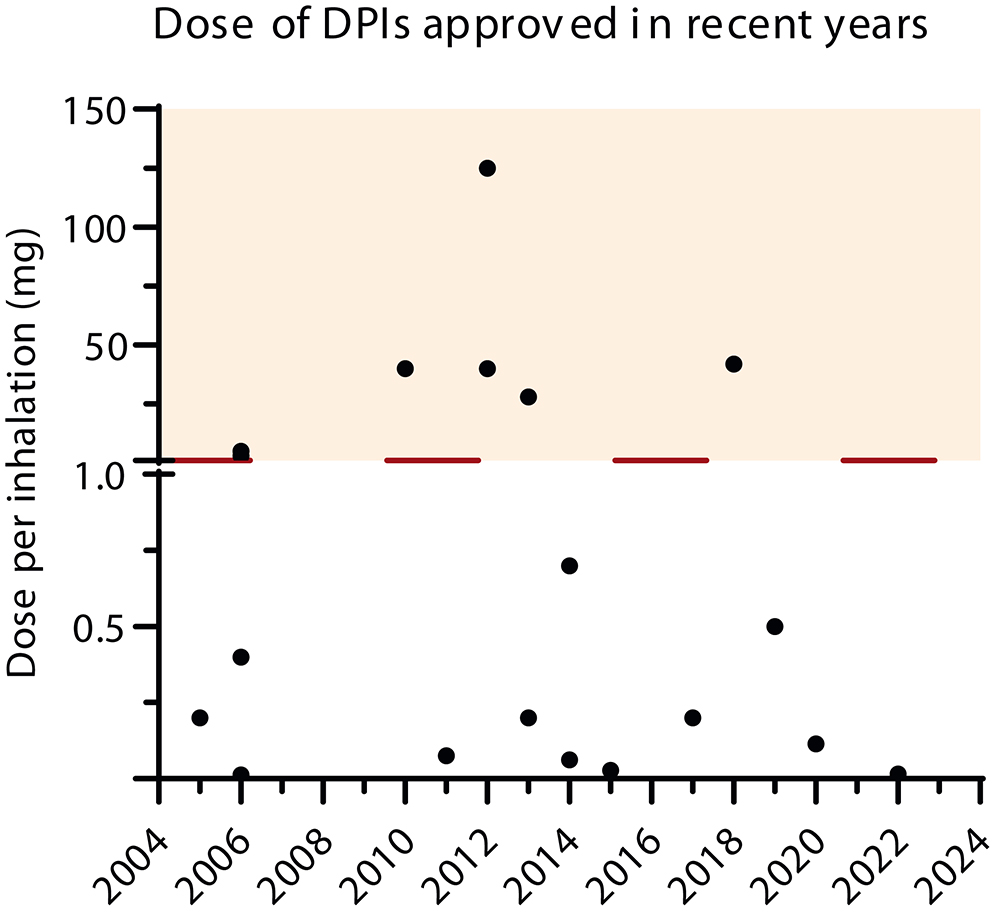
Figure 1. Dose of DPIs approved in recent years.2
High concentrations of drug at the site of action could enable a reduction in dose and dosing frequency, and, subsequently, potential side effects.4 Dry powder inhalers (DPIs) are frequently employed delivery systems for the pulmonary administration of high drug loads of APIs. The majority of DPIs are developed as carrier-based mixtures in which a coarse inert carrier is mixed with the micronised drug substance, with a particle size in the respirable range (<5 μm). Blending the API with an excipient that acts as a carrier improves the aerodynamic performance for low API loads but, as excipient-API interaction is saturated, API-API interaction may result in aerodynamic performance variability.5
Carrier-free dry powder formulations for inhalation are widespread solutions for overcoming these challenges and efficiently deliver the API to the lungs. Particle engineering by spray drying of high load formulations from a solution, can result in a product with adequate performance; however, it often necessitates more restrictive storage and handling conditions due to the amorphous state of API and excipients. Additionally, to successfully stabilise the API amorphous form, relatively high loads of stabilising excipients, such as glass-forming sugars, may be required, reducing the drug product potency. Further, depending on the quality target product profile, namely the dose and dosing frequency, crystalline formulations may be required to avoid a fast release and clearance, as observed in amorphous counterparts.
“Manufacturing methods aimed at achieving consistent particle size distribution for crystalline drugs entail a diverse array of approaches.”
Developing a crystalline formulation with enhanced aerodynamic performance can present a challenge because there is a greater dependency on the physical characteristics and performance of the API. To overcome this challenge, a formulation platform was developed by Hovione (WO2011131947A). This platform technology enables the wet micronisation of the API to the target particle size distribution and the addition of a force control agent to the API suspension, aiming to improve the aerodynamic performance of the drug product, even when the drug load is high (>80% w/w).
There are several force control agents that can be selected, depending on the API characteristics and desired performance. The main cohesion mechanisms and forces of spray-dried particles that impact powder cohesion and flowability are derived from van der Waals forces, electrostatic forces and capillary forces, if there is humidity present. Force control agents (FCAs) can act as powder dispersibility modulators by changing the particles’ surface and consequently impacting van der Waals forces and cohesion.6
Some examples of force control agents that can be used are distearoylphosphatidylcholine (DSPC), dipalmitoyl phosphatidylcholine (DPPC), lecithin or leucine. The force control agent most suitable for a certain product will be dependent on API properties as well as on the desired critical quality attributes (CQAs), namely intended emitted dose and fine particle fraction (FPF).
WET COAT MANUFACTURING CONSIDERATIONS
Crystalline drugs are often used in inhalation formulations owing to their unique and key advantages that contribute to the effective development of safe therapies for the treatment of respiratory diseases and systemic conditions.
When developing crystalline inhalation products, the challenge faced by manufacturers is often related to obtaining uniform crystalline drug particle distribution in a reproducible, controlled and scalable process. Manufacturing methods aimed at achieving consistent particle size distribution for crystalline drugs entail a diverse array of approaches. These include conventional techniques, such as dry or wet milling, categorised as top-down methods, as well as emerging bottom-up strategies, such as controlled crystallisation.
“Formulating a high-load mixture containing crystalline API and a minimal percentage of excipient (applied as a coating on the API surface) enables the development of innovative products, thereby enhancing treatment efficacy.”
Hovione has showcased the efficacy of these techniques in controlling the particle size distribution of crystalline drugs and, subsequently, the aerodynamic performance. Among these, top-down strategies, such as wet polishing, stand out as techniques that are robust and scalable. Although bottom-up approaches, such as controlled crystallisation using micro-fluidisation, have garnered traction, they are still in the early stages of development and face challenges in achieving the target CQAs.7 In that regard, Hovione supports drug product development under quality-by design (QbD) methodologies. Wet polishing integrates a wet media size-reduction technique coupled with spray drying as the isolation step.
A robust process development involves the early identification of potential critical process parameters (pCPPs) and potential CQAs. The development strategy encompasses a laboratory-scale familiarisation phase, which includes an exploration of the process design space leveraged by Hovione’s modelling capabilities. Experimental designs are conducted on the identified pCPPs, establishing correlations between scale-independent variables, CQAs and process performance to facilitate scale-up. Several scales are available at Hovione’s portfolio enabling wet micronisation rates from 24 to 300 L/h. The process pressure: hydraulic pressure ratio can be adjusted based on early development studies as part of the QbD methodology to ensure the achievement of target CQAs.
Wet polishing is the particle engineering technology of choice for drug-alone formulations; however, depending on the API surface chemistry, wet polishing alone may not be sufficient to achieve the desired aerodynamic performance. Through this technology, it is evident that formulating a high-load mixture containing crystalline API and a minimal percentage of excipient (applied as a coating on the API surface) enables the development of innovative products, thereby enhancing treatment efficacy. The surface coating is achieved by dissolving the force control agent in the micronised drug suspension and spray drying the resulting feedstock, thereby promoting the coating and isolation of the API particles. During this stage, the models for droplet and particle formation, alongside the development of both the size-reduction and the spray-drying design spaces, are pivotal in determining the optimal coating of the micronised drug.
CASE STUDIES
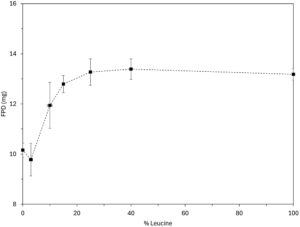
Figure 2. Anti-viral model drug aerodynamic performance.
Aiming to showcase the wet coating platform potentialities for different drugs and using diverse force control agents, two model drugs were tested. The API models chosen were an antiviral drug and a corticosteroid, both insoluble in water (<1 mg/mL). Log P, the antiviral drug, presented a lower Log P (3.3), when compared with the corticosteroid (3.7). The work performed involved the comparison of the API alone with two formulations with increased percentages of a force control agent.
Anti-Viral Model Drug
The anti-viral model drug was suspended in water and wet milled until the target particle size distribution was obtained. Then, the feedstock was fed to a lab-scale spray dryer (Büchi, model B-290) while stirring. On specific formulations, leucine (as FCA) was added to the suspension at increasing relative proportion (Table 1). All formulations showed Dv50 below 3 μm. The aerodynamic performance results obtained by a fast screening impactor (FSI), using an RS01 (Berry / Plastiape) device (100 L/min at 4 kPa), demonstrate that the fine particle dose (FPD) increases with the FCA percentage in the formulation composition until a plateau is achieved (around 25% w/w FCA for this drug). Figure 2 shows a FPD upgrade from 10 to 13 mg as a result of the coating of the crystalline API.
Trials | A | B | C | D | E | F | G |
API (% w/w) | 100 | 97 | 90 | 85 | 75 | 60 | 0 |
Leucine (% w/w) | 0 | 3 | 10 | 15 | 25 | 40 | 100 |
Table 1: Composition of the anti-viral model drug formulations.
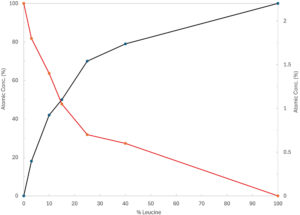
Figure 3. XPS data (red line corresponds to the crystalline drug element P and black line to the leucine’s element C).
To further understand the coating effectiveness of the crystalline drug particles by the force control agent, an X-ray photoelectron spectroscopy (XPS) “fingerprint” model was developed (Figure 3). The presence of the element phosphorous (P) in red indicates the presence of API at the particle surface, as this element is exclusive to this material. The excipient element carbon (C) (black line in Figure 3) was identified through a Hovione “fingerprint” model. The results showed that there is an inflection point at approximately 25% w/w of leucine, where the leucine’s “fingerprint” C element becomes more prominent than the P element from the crystalline drug. These results indicate that at this excipient concentration the API becomes covered by the force control agent leading to an optimal formulation aerodynamic performance.
Corticosteroid Model Drug
In this case study, the corticosteroid was suspended in water and micronised until the target particle size was obtained. After this particle size reduction step, the 5% w/w of force control agents lecithin and DSPC were dissolved in the suspension of Trial 1 and Trial 2, respectively. The suspensions were then fed to a lab-scale spray dryer (Büchi, model B-290) while stirring. All spray-drying trials were characterised for crystalline state of API, geometric particle size by dry dispersion (Sympatec, Basel, Switzerland) and assay by high-performance liquid chromatography. Aerodynamic performance was characterised by FSI, using a RS01 Berry / Plastiape device (100 L/min at 4 kPa). Comparing with the drug alone FSI data, it can be concluded that the wet coat platform led to at least a twofold increase in FPD (mg), for both formulations tested, while maintaining the API crystallinity (Figure 4).
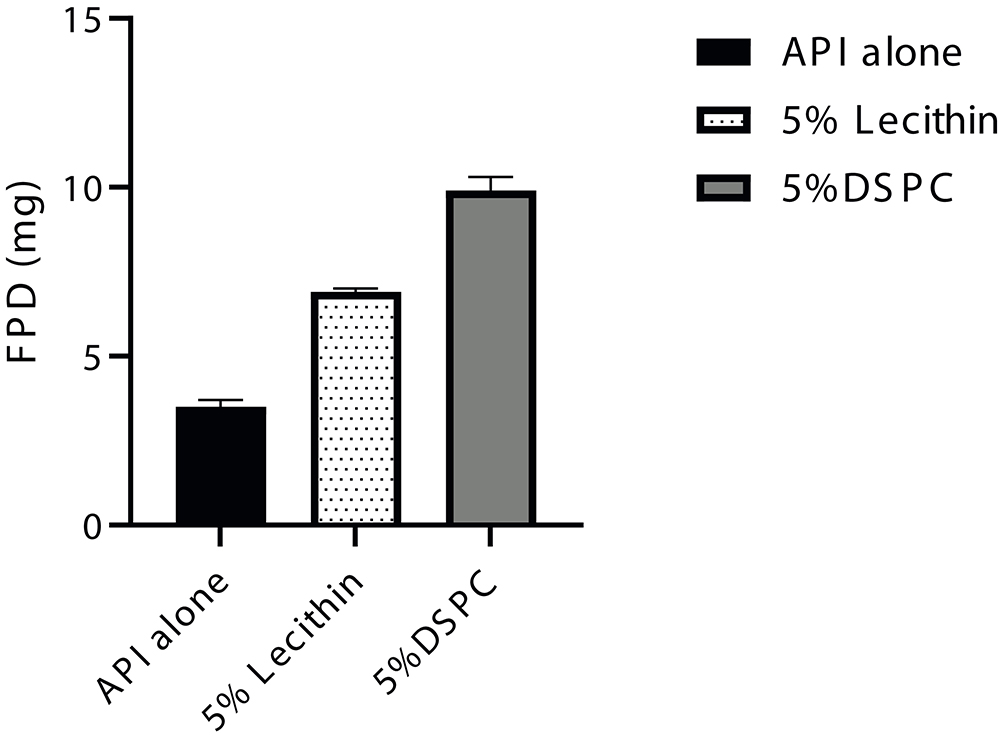
Figure 4. Corticosteroid model drug aerodynamic performance.
CONCLUSIONS
In conclusion, the studies show that the wet coat platform is suitable for improving the efficiency of drug delivery to the lungs, by improving the FPD and, consequently, FPF. This formulation development approach allows the delivery of high payloads while maintaining the crystallinity of the API. This formulation platform presents a significant advantage in terms of dissolution modulation because crystalline particles present a distinct dissolution profile when comparing with amorphous particles. Additionally, the long-term stability of the dry powder is also improved as crystalline particles are known to be physically more stable than their amorphous counterpart. The force control agents added to the formulation could also be changed and optimised as needed to overcome the aerodynamic performance challenges associated with the different APIs’ surface characteristics. As such, Hovione channels its extensive expertise in particle engineering and drug product formulation strategies, positioning itself as a prominent leader in this technology, which seamlessly integrates with wet polishing, optimising the aerosolisation of crystalline drugs.
To learn more from Hovione’s expertise in inhaled and nasal drug development, visit: www.hovione.com/products-and-services/contract-manufacturing-services/drug-product/inhalation-and-nasal.
REFERENCES
- Ye Y, Ma Y, Zhu J, “The future of dry powder inhaled therapy: Promising or Discouraging for systemic disorders?”. Int J Pharm, 2022, Vol 614, p 121457.
- Costa E et al, “Addressing challenges of high dose dry powder delivery: Formulation and Device considerations”. Respiratory Drug Delivery, 2023, Vol 2023, pp 101–112.
- Sibum I et al, “Challenges for pulmonary delivery of high powder doses”. Int J Pharm, 2018, Vol 548(1), pp 325–336.
- Anderson S et al, “Inhaled Medicines: Past, Present, and Future”. Pharmacol Rev, 2022, Vol 74(1), pp 48–118.
- Abiona O et al, “The Optimisation of Carrier Selection in Dry Powder Inhaler Formulation and the Role of Surface Energetics”. Biomedicines, 2022, Vol, 10(11), p 2707.
- Weiler C et al, “Force control and powder dispersibility of spray dried particles for inhalation”. J Pharm Sci, 2010, Vol 99(1), pp 303–16.
- Porfirio T et al, “Controlled crystallisation through microfluidisation as an alternative particle engineering technology for inhalation products”. AIChE Annual Meeting, 2023.