To Issue 144
Citation: Villar G, Miller E, “The Next Generation of Microneedles: Harnessing a Billion Years of Evolution”. ONdrugDelivery, Issue 144 (Mar/Apr 2023), pp 5–9.
Gabriel Villar and Ethan Miller consider examples of nature’s solutions to four challenges faced by microneedles for drug delivery. For each challenge, the authors explain how a natural organism has tackled it and what lessons may be directly applied to microneedle design.
THE PROBLEM WITH NEEDLES
Many people are afraid of needles, with a significant number experiencing such an aversion to them that they will avoid seeking medical care for fear of injection.1 The invention of a needle so thin and short that it is barely visible to the naked eye, called a microneedle, would be welcomed by the needle-phobic and bring other notable benefits, such as near-painless injections, easy self-administration and the freedom to dispose of the device with a negligible sharps risk. Furthermore, such a device would be ideally suited to the administration of drugs into the skin, which may advantageously induce a greater immune response when administering vaccines2 and avoids first-pass metabolism.3,4 These benefits are the driving force behind the predicted growth of the microneedle transdermal drug delivery market from US$5.6 billion (£4.6 billion) in 2020 to $8.0 billion by 2028.5
“An ever-bountiful source of solutions and inspiration is to be found in the natural world, in which ruthless evolution over millions of years has created countless necessarily elegant technical solutions to many problems.”
R&D EFFORTS ARE WELL UNDERWAY
These motivating factors have resulted in the investment of a great deal of effort into the development of microneedle-based drug delivery devices.6 Most are one of four types (solid, coated, hollow or dissolving), but many less-common variants have been investigated.
However, only a small number of therapeutic microneedle products have made it to market so far,7 such as the Soluvia™ injector by BD and the MicronJet™ by NanoPass (Nes Ziona, Israel), that can deliver consistent and painless intradermal injections of drugs and vaccines.8 Sanofi’s IDflu®/Intanza® has also been commercialised worldwide for the delivery of influenza vaccines.8
A growing number of microneedle technologies for the delivery of drugs and vaccines are in clinical trials. For example, PharmaTher (Toronto, Canada) and TSRL Inc (MI, US) are co-developing a microneedle patch to deliver psychedelics and antivirals;9 Radius Health (MA, US) and Kindeva Drug Delivery are evaluating a solid microneedle device for osteoporosis;10 and Micron Biomedical is running a clinical trial for a measles-rubella vaccine administered via microneedles.11
MANY CHALLENGES REMAIN
The novelty of microneedles brings many challenges and, despite good progress, some of these remain to be overcome before this technology sees widespread adoption for drug delivery. The spectrum of challenges has been well documented elsewhere,6 and ranges from difficulties in reproducible manufacturing to ensuring reliable delivery of a complete dose.
The first step to solving most problems is to consider whether they may have been solved already. Even if no prior solutions are a perfect fit, some may be readily adaptable to the present challenge or serve as inspiration to resolve the issue in a drastically different way. An ever-bountiful source of solutions and inspiration is to be found in the natural world, in which ruthless evolution over millions of years has created countless necessarily elegant technical solutions to many problems.
Penetration
Through natural selection, mosquitoes have developed a sophisticated apparatus to effectively and painlessly penetrate the skin of animals and humans to feed on their blood.12 This apparatus consists of a bundle, or “fascicle”, of fine tools called “stylets” that are housed within the mosquito’s proboscis. Together, these stylets comprise a natural microneedle 2 mm long and a mere 20 μm in diameter.13 But how does the mosquito use such a delicate structure to penetrate the skin? The answer is partly in a mechanical feature, and partly in how the fascicle is deployed.
First, the structure has micro-serrations that reduce the contact area between the fascicle and skin tissue, which significantly lowers insertion forces (Figure 1). Studies have found that a living mosquito fascicle requires an insertion force of around 16 μN, which is approximately one thousandth of the force required to insert synthetic ultra-sharp microneedles.14
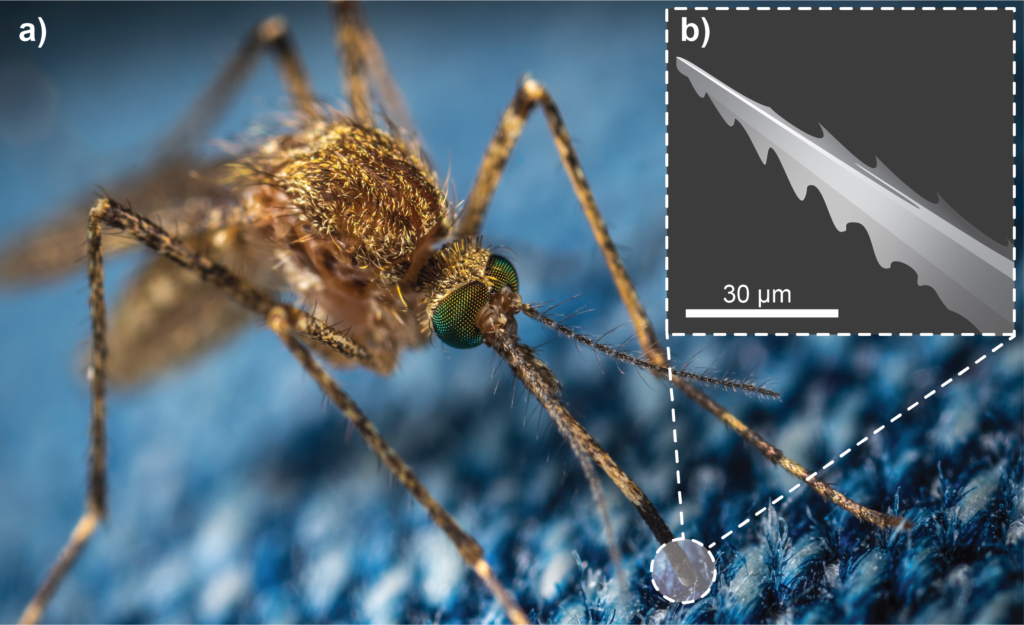
Figure 1: (A) A mosquito uses specialised microneedles in its mouthparts to painlessly bite through animal skin.12 (B) An illustration of a mosquito fascicle, revealing a micro-serrated edge used to cut through skin tissue.13
Second, mosquitoes deploy the fascicle in a way that makes good use of those serrations. Having probed for an ideal spot to penetrate, the mosquito anchors its fascicle onto the skin, then oscillates its head back and forth in a sawing motion to drive the fascicle through the skin. Even this sawing motion is carefully modulated to optimise penetration, starting at 17 Hz and slowing to 6 Hz as the needle is driven deeper.13 Using finite element method (FEM) simulation and skin-penetration models,15,16 researchers have already started to develop mosquito-inspired microneedles that have serrated tips and use co-operative vibration to reduce skin-penetration forces. However, such features have yet to be exploited clinically.
Fracture
Not all microneedles in nature have developed to be painless. The caterpillar Parasa consocia is covered in hollow microneedle spines that pierce any would-be predators and inject a pain-inducing venom. They feature several intriguing microstructures, including a tapered geometry, a side-open tip, a barbed surface and neck-like grooves; but perhaps the most intriguing feature is a subtle one.
Because they are so fine, microneedles can be particularly susceptible to bending and compressive buckling failure. Where the mosquito’s fascicle uses serration and vibration to avoid buckling, the caterpillar’s spine employs a different strategy. The magnitude of buckling is directly related to the Young’s modulus of the material,17 and both scanning electron microscope (SEM) imaging and atomic force spectroscopy have revealed that the Young’s modulus of each caterpillar spine increases progressively from base to tip (Figure 2).18 This gradated material property, together with the geometry of the spine, means that the spines can withstand compressive buckling of up to 3 mN before fracture, which is an order of magnitude greater than the insertion forces required for these ultra-sharp needles to penetrate animal skin.18 Applying these lessons to synthetic microneedles may make them more mechanically robust to a common mode of failure.
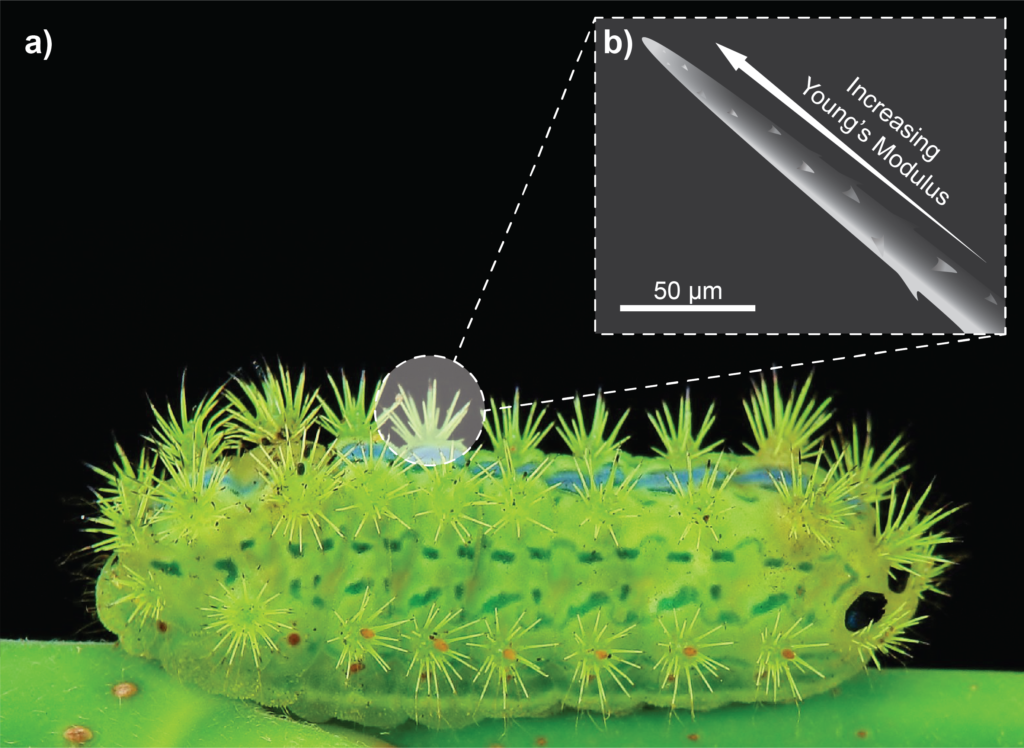
Figure 2: A Parasa conoscia caterpillar with venomous spines robust against mechanical failure (a). Illustration of the microstructure of the spines, in which Young’s modulus increases towards the tip to prevent buckling and bending under compressive stress (b).
Adhesion
Some medical conditions require not a single, rapid injection but a gradual release of a therapeutic agent over time, and microneedle patches are a promising vehicle for such sustained release.19 However, it can be difficult to ensure that a microneedle patch remains attached to the tissue, especially in regions of skin that are subject to large motions. Here, too, one may draw inspiration from nature.
Recently, a microneedle array was developed that was designed to adhere to the user using two different mechanisms, each inspired by a different animal (Figure 3).20 First, each microneedle was surrounded by microscale suction cups, which were modelled on the suckers on octopus tentacles. Second, the suction cups and microneedles were made of a poly-dopamine hydrogel inspired by the composition of the filaments, called “byssi”, that mussels use to attach to a range of surfaces. This improved adhesion, achieved without the use of medical adhesive tape, could make microneedle patches a more viable option for the sustained release of drugs to mobile parts of the body, such as on knee joints to deliver therapeutics for osteoarthritis.23
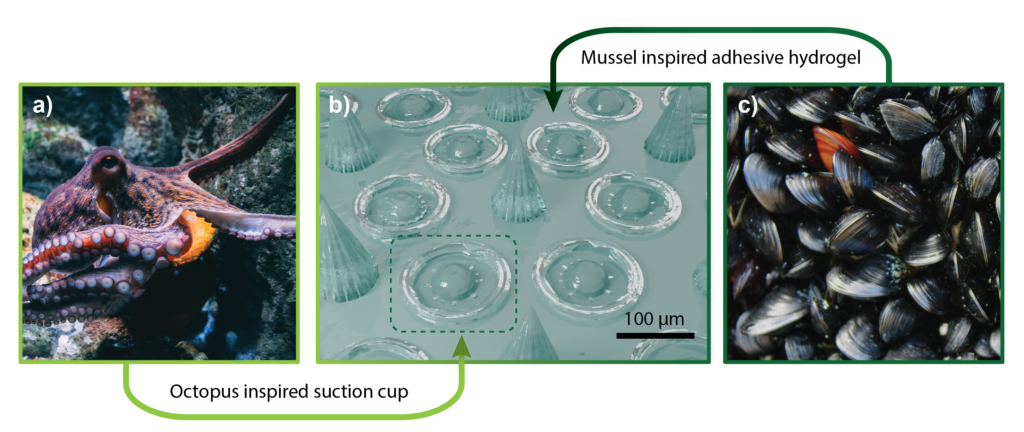
Figure 3: Array of microneedles (centre) with bio-inspired adhesive abilities.20 Each microneedle is surrounded by suction cups inspired by octopus tentacle suckers (left),21 and composed of an adhesive poly-dopamine hydrogel inspired by the filaments used by mussels to adhere to rocks (right).22
Delivery
It is generally difficult to ensure that a microneedle system delivers a complete dose of a liquid drug formulation, especially if the viscosity and surface energy of the formulation have not been carefully engineered for use with microneedles. Fortunately, there already exists a solution that delivers a liquid formulation rapidly and reliably into animal tissue.
In a collection of species known as “rear-fanged” snakes, some of their fangs are not hollow but instead feature a groove that runs along the fang’s surface (Figure 4). When the snake bites into an animal, venom runs down the capillary channel formed between the groove and the unfortunate animal’s tissue. Understanding how this flow is driven by surface tension has allowed researchers to exploit the same effect, and further optimise it by designing synthetic microneedle “fangs” with multiple grooves on each.26 The use of solid rather than hollow microneedles for the delivery of liquid drugs improves the mechanical strength of the needles and simplifies their manufacture. The technology shows promise for the delivery of fast vaccinations, being capable of inducing a robust immune response from a single administration.26
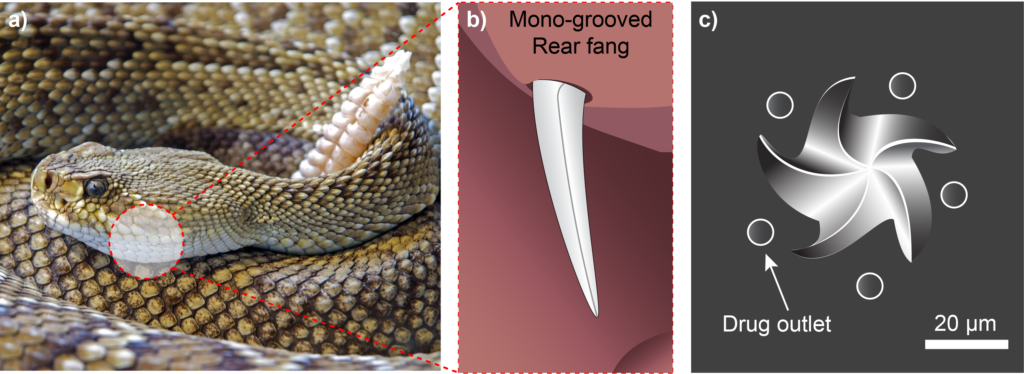
Figure 4: (A) Rear-fanged snake25 with (B) mono-grooved fangs to aid delivery of venom to prey.25 (C) Snake fang multi-grooved solid microneedles, optimised for delivery of liquid formulations.26
CONCLUSION
The development of a novel, successful medical device is typically a hugely ambitious undertaking. A concept that seems promising at first may fall at any number of hurdles on its way to launch; it may be let down by material properties, variability in manufacturing, reaction to sterilisation or subtle design issues that only manifest in late-stage testing. Leveraging existing solutions to closely related problems elsewhere, whether in industry or nature, can dramatically speed up product development. Technology scouting is an essential part of rapid innovation – by avoiding reinventing the wheel, it frees up resources for investment where they can have the greatest impact and reduces the time to market for solutions that patients urgently need.
REFERENCES
- Love AS, Love RJ, “Considering Needle Phobia among Adult Patients During Mass COVID- 19 Vaccinations”. J Prim Care Community Health, 2021, Vol 12, Article 21501327211007393.
- Yang J et al, “Recent advances of microneedles used towards stimuli-responsive drug delivery, disease theranostics, and bioinspired applications”. Chem Eng J, 2021, Vol 426, Article 130561.
- Aldawood FK, Andar A, Desai S, “A Comprehensive Review of Microneedles: Types, Materials, Processes, Characterizations and Applications”. Polymers (Basel), 2021, Vol 13(16), Article 2815.
- Jung JH, Jin SG, “Microneedle for transdermal drug delivery: current trends and fabrication”. J Pharm Investig, 2021, Vol 51(5), pp 503–517.
- “Microneedle Drug Delivery Systems Market Size, Share & Trends Analysis Report By Type (Hollow, Dissolving, Solid, Coated), By Material (Metal, Silicon, Polymer), By Application, By Region, And Segment Forecasts, 2021 – 2028”. Grand View Research, Oct 2021.
- Avcil M, Çelik A, “Microneedles in Drug Delivery: Progress and Challenges”. Micromachines (Basel), 2021, Vol 12(11), Article 1321.
- Halder J et al, “Microneedle Array: Applications, Recent Advances, and Clinical Pertinence in Transdermal Drug Delivery”. J Pharm Innov, 2021, Vol 16(3), pp 558–565.
- Larrañeta E et al, “Microneedle arrays as transdermal and intradermal drug delivery systems: Materials science, manufacture and commercial development”. Mater Sci Eng R Rep, 2016, Vol 104, pp 1–32.
- “PharmaTher and TSRL Enter into Co-Development Agreement For Microneedle Patch Delivery Technology for Psychedelics and Antivirals”. GlobeNewswire, Jun 2021.
- Miller PD et al, “Phase 1b Evaluation of Abaloparatide Solid Microstructured Transdermal System (Abaloparatide-sMTS) in Postmenopausal Women with Low Bone Mineral Density”. Clin Drug Investig, 2021, Vol 41(3), pp 277–285.
- “Measles and Rubella Vaccine Microneedle Patch Phase 1-2 Age De-escalation Trial”. ClinicalTrials.gov, May 2019.
- Gittard SD et al, “The Effects of Geometry on Skin Penetration and Failure of Polymer Microneedles”. J Adhes Sci Technol, 2013, Vol 27(3), pp 227–243.
- Ma G, Wu C, “Microneedle, bio-microneedle and bio-inspired microneedle: A review”. J Control Release, 2017, Vol 251, pp 11–23.
- Roxhed et al, “Penetration-Enhanced Ultrasharp Microneedles and Prediction on Skin Interaction for Efficient Transdermal Drug Delivery”. J Microelectromech Syst, 2007, Vol 16(6), pp 1429–1440.
- Aoyagi S, Izumi H, Fukuda M, “Biodegradable polymer needle with various tip angles and consideration on insertion mechanism of mosquito’s proboscis”. Sens Actuator A Phys, 2008, Vol 143(1), pp 20–28.
- Izumi H et al, “Realistic imitation of mosquito’s proboscis: Electrochemically etched sharp and jagged needles and their cooperative inserting motion”. Sens Actuator A Phys, 2011, Vol 165(1) pp 115–123.
- Radhika C, Gnanavel BK, “Buckling analysis of polymer microneedle for transdermal drug delivery”. Mater Today Proc, 2021, Vol 46(9), pp 3538–3541.
- Ma GJ, Shi LT, Wu CW, “Biomechanical Property of a Natural Microneedle: The Caterpillar Spine”. J Med Devices, 2011, Vol 5(3), Article 034502.
- May M, “Why drug delivery is the key to new medicines”. Nat Med, 2022, Vol 28, pp 1100–1102.
- Zhang X et al, “Bioinspired Adhesive and Antibacterial Microneedles for Versatile Transdermal Drug Delivery”. Research (Wash DC), 2020, Vol 2020, Article 3672120.
- Picchiottino D, “Brown and black dragon in water”. Unsplash, Aug 2021.
- Castille A, “Black and red ladybug on white textile”. Unsplash, Sep 2020.
- Zhou P et al, “Strategy for osteoarthritis therapy: Improved the delivery of triptolide using liposome-loaded dissolving microneedle arrays”. Int J Pharm, 2021, Vol 609, Article 121211.
- Dixon AR, Vondra I “Biting Innovations of Mosquito-Based Biomaterials and Medical Devices”. Materials (Basel), 2022, Vol 15(13), Article 4587.
- “Diamond Back Rattle Snake”. Pixabay, May 2016.
- Bae W-G et al, “Snake fang-inspired stamping patch for transdermal delivery of liquid formulations”. Sci Transl Med, 2019, Vol 11(503), Article eaaw3329.