To Issue 158
Citation: Fenn M, Brooks Clair, “Better in Vivo Prediction From OINDP Testing: a Goal Worth Pursuing?”. ONdrugDelivery, Issue 158 (Apr 2024), pp 36–40.
Matthew Fenn and Clair Brooks, consider the motivation for adopting more clinically representative test set-ups in orally inhaled and nasal drug product development and, more specifically, for the demonstration of bioequivalence, as well as the practicalities of doing so and the tools available to support such efforts.
As recently released information from the US Pharmacopeia (USP) Expert Panel on New Advancements in Product Performance Testing (EP-NAPPT) indicates, there is scope for metrics for orally inhaled and nasal drug products (OINDPs) measured using compendial methods to become more clinically meaningful – if how predictive they are of in vivo lung delivery can be improved.1 This observation highlights some important issues for the OINDP community, for both innovator and generic product development. For example, how easily can the clinical relevance of established test methods be improved? And what value is there in doing so if such testing is not yet required by regulators?
“Relative to in vivo testing, in vitro methods are faster, less expensive and more reproducible.”
Relative to in vivo testing, in vitro methods are faster, less expensive and more reproducible. Enhancing them to better support in silico modelling, and to minimise reliance on pharmacokinetic (PK) and pharmacodynamic (PD) studies, could therefore be highly beneficial.
ASSESSING THE POTENTIAL BENEFITS OF BETTER IN VITRO IN VIVO CORRELATIONS
Regulatory guidance calls for in vitro testing for extensive product characterisation, including the measurement of critical quality attributes (CQAs) such as delivered dose uniformity (DDU) and aerodynamic particle size distribution (APSD).2,3 Implemented under standardised, well-controlled conditions, in vitro tests are simple, easy to validate and low cost, relative to in vivo testing. However, robust in vitro–in vivo correlations (IVIVCs) are scarce for OINDPs, in large part due to the impact of patient-to-patient variability with respect to technique, physiology and disease state/lung impairment.
While in vitro studies are also required for all NDAs, the situation with regards to accelerated NDAs (ANDAs) is more nuanced. The EMA proposes a “stepwise” approach to generic submissions that allows for acceptance on the basis of in vitro data alone, provided that demanding criteria are met.4 Recent guidance has reiterated this approach for the specific case of metered dose inhaler (MDI) reformulation with lower global warming potential (GWP) propellants.5
In contrast, the US FDA’s 505(j) and 5050(b)(2) pathways used for generics and super generics respectively call for a “weight of evidence” approach, involving PK (comparative systemic exposure studies) and PD studies, alongside in vitro testing (Figure 1). That said, the FDA has stated that it is openly supportive of “novel bioequivalence (BE) approaches”,6 and there are a growing number of product-specific guidances that include routes to approval via in vitro data alone, such as fluticasone propionate and mometasone furoate nasal spray,7,8 or without PD studies, such as beclomethasone dipropionate metered aerosol.6
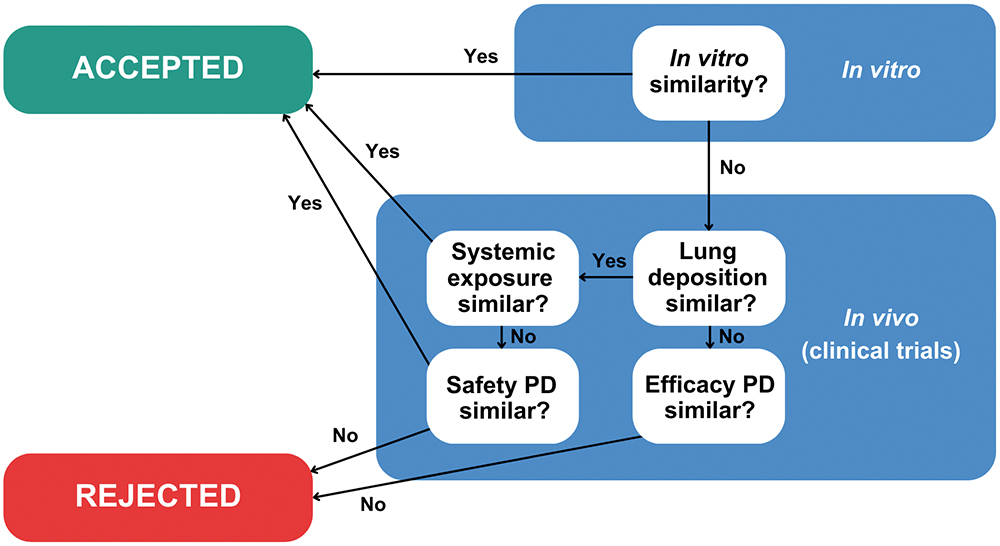
Figure 1: The EMA’s stepwise approach for ANDA submissions differs from the FDA’s weight of evidence assessment.
“Though most regulatory submissions will undoubtedly continue to rely on both compendial methods and in vivo data, better IVIVCs could shift the balance with respect to the extent to which they do so, especially for ANDAs.”
Against this backdrop, the motivations for improving the clinical relevance of in vitro methods come into focus. For both ANDAs and NDAs, better IVIVCs would facilitate a Quality by Design (QbD) approach to development, making it easier to identify CQAs and knowledgeably manipulate formulation and device towards clinical success. Design of experiment (DoE) studies, such as that reported by Lock et al to develop enhanced description of the performance of the dry powder inhaler (DPI) Breo® Ellipta™ (fluticasone furoate and vilanterol, GSK), exemplify this approach.9 On the other hand, for ANDA submissions, better IVIVCs equate with the ability to robustly confirm BE in vitro, increasing the likelihood of approval via in vitro routes alone and reducing reliance on in vivo data.
This last point is especially important, as clinical studies have well-recognised limitations. The therapeutic relevance of PK studies is questionable for OINDPs targeting local efficacy as opposed to systemic action; although by reliably confirming equivalence with respect to total systemic bioavailability, test and reference, they do support claims of equivalent safety. PD studies, on the other hand, are challenging to implement due to the need to work with a diseased, and consequently variable, patient population and to identify a suitable biomarker; high variability and poor sensitivity are both commonplace. Moving away from clinical endpoint PD studies for ANDAs is therefore highly desirable.
In summary, although most regulatory submissions will undoubtedly continue to rely on both compendial methods and in vivo data, better IVIVCs could shift the balance with respect to the extent to which they do so, especially for ANDAs. Potential gains include faster development, better clinical study design and interpretation and, ultimately, a lower risk of failure in the clinic.
BUILDING ON COMPENDIAL METHODS
In emphasising IVIVCs, it is helpful to acknowledge that this represents a divergence in intent from compendial methods. These were established to safeguard the quality of pharmaceutical products, so their development therefore prioritised simplicity, repeatability, broad applicability and sensitivity, rather than specific clinical exactness.
The gap analysis carried out by the EP-NAPPT highlights several areas where there is scope for productive modification of compendial methods to accelerate product development and provide supplementary data to strengthen submission packages, pointing to the potential benefits of using:1
- More realistic mouth-throat models and inspiratory flow profiles to make APSD measurement more representative of in vivo delivery to the lung
- More realistic nasal inlets and air flow rates to make assessments of off-target drug delivery – pulmonary as opposed to intranasal – more reliable
- Nasal casts to assess regional deposition within the nasal cavity
- In vivo-relevant dissolution testing to provide a better understanding of local and systemic exposure in both the nose and lung
- Morphologically directed Raman spectroscopy (MDRS) to measure drug-specific particle size distribution data for “as delivered” nasal suspensions in place of a clinical endpoint study.
The issue of mouth-throat models provides a good example of how, with the right tools, clinical relevance can be improved without compromising the reproducibility and utility of test methods. The European Pharmacopeia (PhEur) and USP induction port specified for APSD measurement of orally inhaled products (OIPs) was developed to provide a robust representation of the throat but clearly lacks physiological realism and is known to underpredict deposition in the upper respiratory tract.10 An anatomically precise throat cast is the obvious alternative, but inter-subject variability, along with the practicalities of manufacture and routine use, are major limitations. Rather, the better option is a mouth-throat model that is simplified as far as possible, within the constraint of realistically reflecting deposition behaviour across a broad patient population, as exemplified by the Alberta Idealised Throat (AIT), which is depicted in Figure 2.
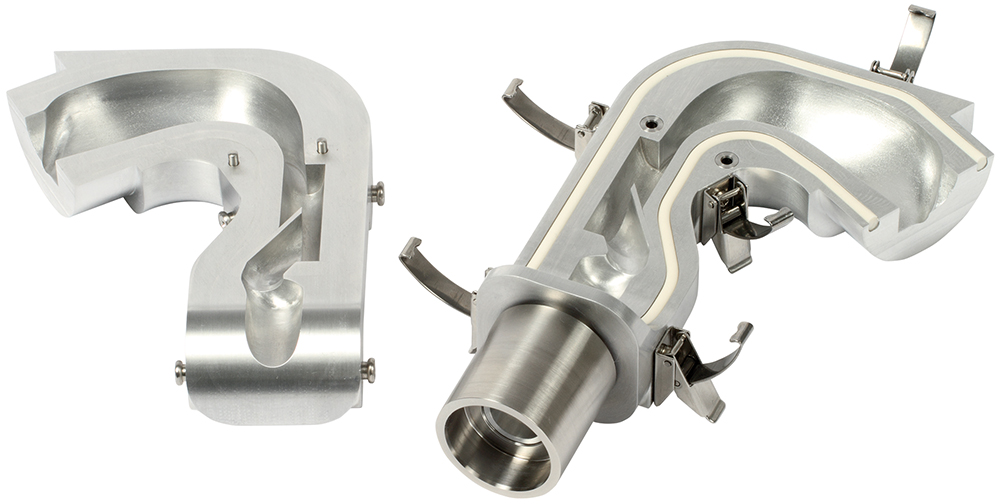
Figure 2: The AIT is an easy-to-use accessory for APSD measurement that provides a more clinically realistic representation of the upper respiratory tract than the standard USP and PhEur induction port.
However, establishing better IVIVCs relies not only on improved representations of patient physiology but also on the assessment of device handling and use characteristics. For example, EMA guidance for the demonstration of BE for MDIs specifically references the “cold freon” effect – the chilling sensation associated with impaction of the aerosol plume at the back of the throat and propellant evaporation. Since this effect can cause a patient to alter, or even abort, an inhalation manoeuvre, parity is highly relevant to the interchangeability of MDI products.4 MDIs are also necessarily tested with facemasks, depending on the target patient population, as detailed in USP <1602>, which covers testing for MDIs with spacers and valved holding chambers.11
As researchers become more aware of the merits of better IVIVCs, the test apparatuses already available to achieve them are becoming better understood, more mainstream and more widely used, making it easier to assess the potential benefits.
PROGRESS TOWARDS BETTER IVIVCS
Investigating the Impact of Inhalation Profile
Since drug delivery with a DPI is driven solely by the inhalation manoeuvre of the patient, in vitro investigations of the impact of different patient inhalation profiles are especially valuable for this class of OIPs. For example, they allow researchers to determine whether or not a specific patient group will receive a clinically effective dose, and whether test and reference products are equally sensitive to patient technique. Figure 3 shows a cascade impactor set-up for an APSD measurement incorporating an AIT, a breathing simulator for application of a specific inhalation profile rather than the square wave associated with compendial methods and a mixing inlet to simultaneously maintain a constant air flow rate through the cascade impactor. The separation efficiency of cascade impactors is a function of air flow rate, which must be known and constant for reliable measurement.
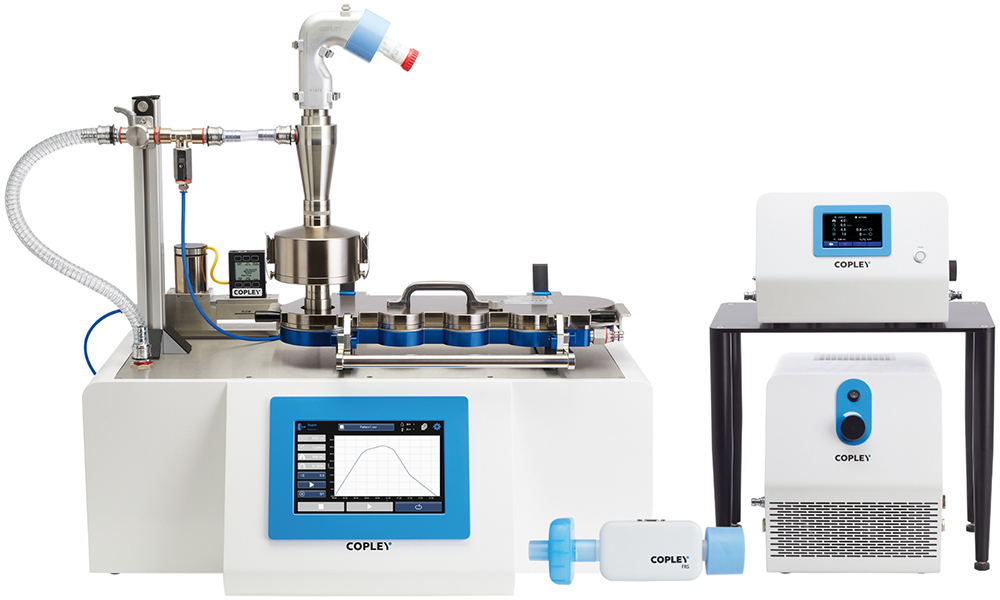
Figure 3: A test set-up for clinically representative DPI testing complete with breathing simulator, mixing inlet and AIT.
Work by Abadelah et al to assess the impact of inhalation profile on drug delivery with an Onbrez Breezhaler® (indacaterol maleate, Novartis) exemplifies the studies that these set-ups can enable.12 Inhalation profiles of patients with chronic obstructive pulmonary disease (COPD) were quantified in terms of peak inspiratory flow (PIF), inhalation volume and initial acceleration rate as they inhaled through an empty Breezhaler in accordance with instructions in the patient information leaflet. APSD measurements were then taken using an IVIVC test set-up and the resulting patient inhalation profiles. Total emitted dose (TED), fine particle dose (FPD) and mass median aerodynamic diameter (MMAD) were all found to be dependent on PIF and inhalation volume, with the most unproductive profiles producing a TED of 61% of the nominal dose and an FPD of just 19%.
Interestingly, a comparable study of the performance of Breezhaler with a once-daily long-acting muscarinic antagonist (LAMA) glycopyrronium formulation indicated more robust performance with higher TED and FPD values and less variability across inhalation profiles representative of COPD patients.13 Such results underline the formulation- and product-specific nature of flow-rate sensitivity and the corresponding need for testing.
“The buoyancy of the OINDP marketplace is stimulating both interest and innovation in testing practice.”
For generics, analogous testing is helpful in demonstrating equivalence with respect to device handling and robustness to patient physiology, as exemplified by work from Shepherd et al in assessing the performance of Wixela Inhub® (fluticasone propionate and salmeterol, Mylan Pharmaceuticals), a generic version of Advair Diskus (fluticasone propionate and salmeterol, GSK).14 The Wixela Inhub was shown to exhibit low flow rate dependency, delivering a relatively consistent dose across inhalation profiles representative of COPD patients and, crucially, to deliver comparable performance to Advair Diskus in this regard (as assessed from published data). Similarly, Chrystyn et al investigated the performance of DuoResp Spiromax® (budesonide and formoterol, Teva Pharmaceuticals) relative to Symbicort Turbuhaler® (budesonide and formoterol, AstraZeneca), finding Spiromax to be more robust with respect to inhalation profile but more sensitive to mouth-throat geometry.15
As noted, DPIs are typically the focus of studies into the impact of the inhalation profile, but there is also evidence that nebuliser testing may benefit from similar refinement. Svensson et al found that using a breathing simulator and mixing inlet to apply a patient-representative breathing pattern across a nebuliser during APSD measurement resulted in a fine droplet dose equal to only 72% of that obtained with compendial methods; a key conclusion being that a modified impactor set-up might be preferable for clinical programme support.16
Clinically Relevant Assessment of the Safety of Nasal Drug Products
The requirement for nasal inlets to assess deposition in the nasal cavity and to measure off-target deposition in the lungs is analogous to the need for more realistic mouth-throat models, and comparable solutions are evolving. For example, the same team that developed the AIT has designed the Alberta Idealised Nasal Inlet (AINI), shown in Figure 4, an accessory that is similarly easy to use and representative of a broad patient population. Using the AINI in a cascade impactor test setup allows researchers to simultaneously investigate regional deposition in the nasal cavity and the risk of pulmonary delivery, an important safety concern for nasal drug products.
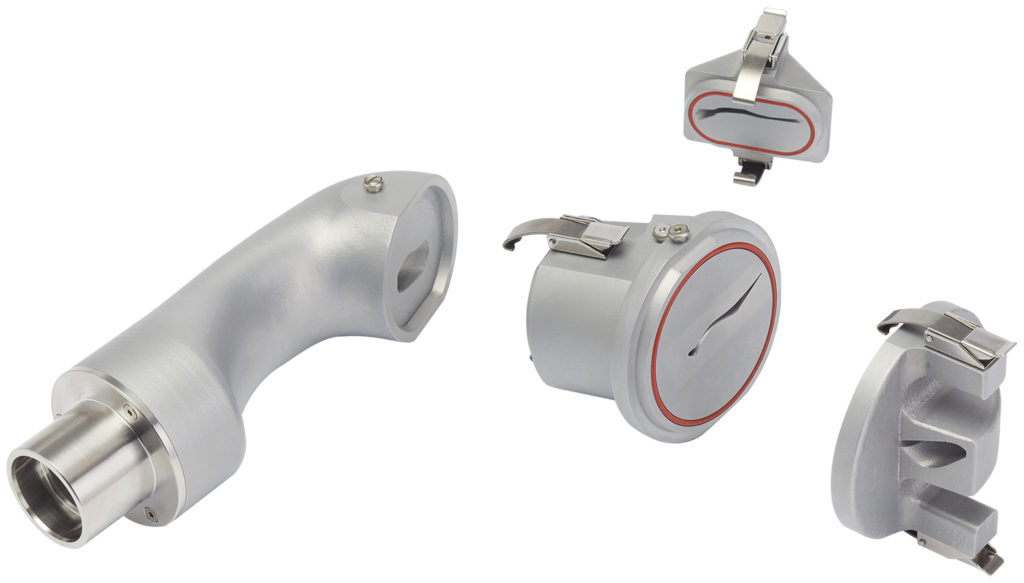
Figure 4: The AINI is a clinically validated accessory for investigating regional deposition in the nasal cavity and the risk of off-target drug delivery to the lungs.
In a study by Potts et al, the AINI was used to determine the <10 μm fraction for three spray-dried caffeine formulations with different particle size characteristics.17 For all three formulations, less than 2.5% of the sample deposited in the impactor, suggesting a minimal safety risk, even though laser diffraction measurements indicated a <10 μm fraction of ~95% for the finest formulation. This important result underlines the value of using a clinically representative nasal inlet in combination with an aerodynamically relevant particle sizing technique to robustly assess the safety of nasal drug products. The particle size of the formulation was also shown to influence regional deposition within the nasal cavity, a potentially important factor for product developers seeking to optimise clinical efficacy.
Nasal drug product testing practice is arguably less well established, relative to that for OIPs, but evolving quickly; interest in the application of inhalation profiles is similarly emerging in the drive to make both deposition and off-target delivery studies as representative as possible. More broadly, the buoyancy of the OINDP marketplace is stimulating both interest and innovation in testing practice. The robust reproducibility and sensitivity of compendial methods is crucial for product quality control, but working towards better IVIVCs is widely recognised as an important goal to strengthen the utility of in vitro methods for product development and regulatory submissions.
To learn more about Copley’s inhaler testing expertise, visit: www.copleyscientific.com/inhaler-testing.
REFERENCES
- Sakagami M, Fotaki N, “Testing the In Vitro Product Performance of Inhalation and Nasal Drug Products: Views of the USP Expert Panel”. Pharmaceutical Forum 48(5), Sep–Nov 2022.
- “Draft Guidance for Industry: Metered Dose Inhaler (MDI) and Dry Powder Inhaler (DPI) Drug Products–Quality Considerations”. US FDA, Apr 2018.
- “Guideline on the Product Quality of Inhalation and Nasal Products”. EMA, Jun 2006.
- “Guideline on the requirements for clinical documentation for orally inhaled products (OIP) including the requirements for demonstration of therapeutic equivalence between two inhaled products for use in the treatment of asthma and chronic obstructive pulmonary disease (COPD) in adults and for use in the treatment of asthma in children and adolescents”. EMA, Jan 2009.
- “Questions and answers on data requirements when transitioning to low global warming potential (LGWP) propellants in oral pressurised metered dose inhalers”. EMA, Nov 2023.
- “Draft Guidance on Beclomethasone Dipropionate”. US FDA, May 2019.
- “Draft Guidance on Fluticasone Propionate”. US FDA, May 2023.
- “Draft Guidance on Mometasone Furoate”. US FDA, May 2023.
- Lock DJ, Watkins A, Munro A, “DPI Performance Modelling Using an Inhalation Simulator and Oropharyngeal Model: A more Patient-Relevant Approach for Device Development”. Presented at DDL 25, Dec 2014.
- Zhang Y, Gilbertson K, Finlay WH, “In vivo-in vitro comparison of deposition in three mouth-throat models with Qvar and Turbuhaler inhalers”. J Aerosol Med, 2007, Vol 20(3), pp 227–235.
- “<1602> Spacers and Valved Holding Chambers Used with Inhalation Aerosols—Characterization Tests”. United States Pharmacopeia, 2023.
- Abadelah M, Chrystyn H, Larhrib H, “Use of inspiratory profiles from patients with chronic obstructive pulmonary disease (COPD) to investigate drug delivery uniformity and aerodynamic dose emission of indacaterol from a capsule based dry powder inhaler”. Eur J Pharm Sci, 2019, Vol 134, pp 138–144.
- Zanker D et al, “‘In Vitro Dose Delivery Performance of Glycopyrronium using Representative Inspiratory Flow Profiles derived from COPD Patients”. Presented at DDL 23, Dec 2012.
- Shepherd T et al, “In Vitro Performance of the Wixela Inhub Inhaler Using Severe Chronic Obstructive Pulmonary Disease Patient Inhalation Profiles.” J Aerosol Med Pulm Drug Deliv, 2022, Vol 35(3), pp 154–165.
- Chrystyn H et al, “Effect of inhalation profile and throat geometry on predicted lung deposition of budesonide and formoterol (BF) in COPD: An in-vitro comparison of Spiromax with Turbuhaler”. Int J Pharm, 2015, Vol 491(1–2), pp 268–276.
- Svensson M et al, “Laboratory Study Comparing Pharmacopeial Testing of Nebulizers with Evaluation Based on Nephele Mixing Inlet Methodology”. AAPS PharmSciTech, 2018, Vol 19(2), pp 565–572.
- Potts JC et al, “Investigations into the Relationship Between Spray Dried Powder Particle Size and Deposition in Nose and Lung Analogues when Actuated from a Nasal Device”. Resp Drug Deliv, 2023, Vol 2023, pp 431–436.