To Issue 127
Citation: Copley M, Sipitanou A, “Improving in Vitro Test Methods for Nasal Drug Products”. ONdrugDelivery, Issue 127 (Nov 2021), pp 19–24.
Mark Copley and Anna Sipitanou look at how in vitro testing is being refined for greater repeatability and to maximise its value as a low-cost tool for product development, including an assessment of the role of automation in improving the sensitivity of in vitro testing.
“Pharmacokinetic studies have limited relevance when it comes to demonstrating bioequivalence for locally acting products, intensifying the need to optimise the application of in vitro techniques.”
There are sound reasons why in vitro test methods for nasal drug products are currently subject to scrutiny with a view towards their improvement, such as by shaping them to provide more precise information for targeted delivery. The nasal route is of growing interest for systemic drug delivery, in no small part due to the access it provides to the central nervous system and the associated potential to bypass the blood-brain barrier.1 Nasal drug products for migraine already demonstrate this potential, which holds promise for the treatment of neurological conditions such as Alzheimer’s disease, depression and schizophrenia. For therapies such as these, drug delivery to specific areas of the nasal cavity can be important. Nasal vaccine delivery is also now increasingly well-established, offering recognisable advantages for the delivery of vaccines for influenza, HIV and hepatitis.2 Furthermore, trials for covid-19 nasal vaccines are already underway.3
On the other hand, nasal sprays for local action, such as those for the treatment of allergic rhinitis and hay fever, still dominate the market, making them an important target for generic manufacturers. Pharmacokinetic studies have limited relevance when it comes to demonstrating bioequivalence for locally acting products,4,5 intensifying the need to optimise the application of in vitro techniques. Sensitive techniques with proven clinical relevance have a vital role to play in supporting abbreviated new drug applications.
NASAL DRUG DELIVERY – FROM GENERATION TO DEPOSITION
Many commercial nasal drug products are mechanical metered dose sprays, packaged either as a multi- or unit-dose format. Nasal aerosols that employ a propellant for drug delivery are an alternative for liquid formulations, along with nasal drops, which are a popular choice for locally acting therapeutics. Nasal powders have also been commercialised, as exemplified by Baqsimi (glucagon) (Eli Lilly), for the treatment of severe hypoglycemia.6 Closer examination of the mechanisms of drug delivery via nasal spray can help to elucidate the challenges associated with product development, many of which are common to all nasal drug products.
Drug delivery via a nasal spray is driven by a manually actuated metering pump, the performance of which is manipulated via design parameters such as precompression ratio and the length, geometry and orifice size of the actuator. Nasal spray formulations are solutions or suspensions of one or more APIs, often in an aqueous-based system, with precisely controlled physical properties, notably their rheology. The properties of such formulations are optimised for stability and for dispersion by the shear force applied via actuation of the chosen device.
“In vitro test methods generate vital information and are lower cost, less variable, easier to control and more reliably differentiating than
clinical trials.”
The patient is the final variable in the drug delivery process. Factors such as insertion depth into the nostril, orientation angle and the force/time profile applied during actuation all influence the characteristics of the delivered dose. Furthermore, there is the unique physiology of the patient’s nasal cavity to contend with. The vasculature-rich turbinates are the typical target for drug delivery, however, there is the potential for very fine (<10 μm) droplets to penetrate through the nasal cavity and deposit in the lung, compromising drug safety, while larger droplets may deposit at the front of the nose, undermining efficacy. Droplet collection in the anterior cavity and subsequent dripping from the nostril or, conversely, an unpleasant taste after product use due to droplets reaching the nasopharynx, are both unwanted side effects of suboptimal drug deposition.
This brief examination of the factors influencing nasal drug delivery highlights the multifactorial space that must be robustly explored to develop a nasal drug product with consistent, reproducible and well-defined deposition characteristics. In vitro test methods generate vital information and are lower cost, less variable, easier to control and more reliably differentiating than clinical trials. However, they may lack clinical relevance. Scrutiny as to whether the precision, relevance and application of current techniques is optimal is therefore a natural consequence of the desire for faster, more cost-efficient development.
ASSESSING THE PERFORMANCE OF NASAL DRUG PRODUCTS
In vitro tests recommended in US FDA guidance for nasal drug products include:5
- Single actuation content (alternatively referred to as dose uniformity) through the life of the container
- Droplet size distribution by laser diffraction
- Drug in small particles/droplets (for nasal sprays) or particle/droplet size distribution by cascade impaction (for nasal aerosols)
- Drug particle size distribution by microscopy (for suspension formulations)
- Spray pattern
- Plume geometry
- Priming and repriming.
These well-established tests provide a wealth of information to guide product development and/or demonstrate bioequivalence. Dose uniformity testing, for example, confirms the consistency with which the label dose is delivered across the lifetime of the product. Droplet size distribution has a defining influence on in vivo behaviour; droplet sizes in the range of 20–30 μm, in combination with low injection velocities, are associated with high levels of drug deposition in the turbinates,7 and the amount of drug in small particles is relevant to the potential hazard of pulmonary deposition. Priming and repriming tests assess how reliably and consistently the product works if used according to the instructions for use with respect to, for example, storage and shaking.
However, the standard methods and equipment have their limitations, especially when set against efforts to extend the application of nasal drug delivery, in particular with respect to the study of regional deposition. Supplementary techniques deployed for the study of regional deposition behaviour include testing with nasal casts or replicas and gamma scintigraphy, though neither is readily deployed in routine workflows. Important issues relating to the use of realistic nasal replicas include the manufacture of such replicas and the time-consuming, manually intensive nature of drug recovery, due to the complex geometry involved.
Gamma scintigraphy is a non-invasive imaging technique that involves incorporating radioactive tracers into a formulation and subsequently detecting their location, in vivo, following product use. With this technique, it is possible to generate confirmatory data that test and reference product deposits analogously, to support bioequivalence claims and to compare formulations and/or devices with respect to drug delivery to a specific target. However, again, gamma scintigraphy is far from a routine benchtop tool.
When it comes to shaping analytical tools to better support nasal drug product development, it is important to consider both the information being sought and the practicalities of routine measurement.
AUTOMATION AND ITS ROLE IN REDUCING CONFOUNDING VARIABILITY
Automation is a well-recognised route to easing the manual burden associated with routine analysis while simultaneously improving data quality. Just as patient technique is a source of variability in nasal drug delivery, manual spray actuation can erode the sensitivity of in vitro testing. Automating device actuation can therefore play a useful role in reducing variability and improving data integrity, as evidenced by the US Pharmacopeia (USP) specification that “a mechanical means of actuating the pump assembly be employed to deliver doses for collection” when testing nasal sprays.8
Reducing method variability enables better investigation of the variability that is actually of interest to drug developers – the variability that arises from differences relating to the device, formulation or patient. In the same way, reducing method variability improves the sensitivity of comparative studies in generic product development, making it easier to robustly detect differences between a test and reference product, or conversely to confirm equivalence more reliably.
Table 1 shows data from a single-actuation content study carried out using Vertus II, Copley Scientific’s automated system for nasal spray actuation, in combination with Copley’s Nasal Spray Dose Collector (NSDC), an innovative accessory for dose sampling. Crucial to any testing method is the complete and demonstrable capture of the delivered dose. However, sample loss due to dripping and leakage is commonplace, due to the need to fire nasal sprays upwards to simulate actual product use coupled with the widespread use of glass flasks or vials for dose collection.
NSDC ID | DD NSDC (μg) | Nozzle (μg) | MD (μg) | Recovery (MD % of label claim) |
NSDC 1 | 49.909 | 0.224 | 50.13 | 100 |
NSDC 2 | 48.567 | 0.494 | 49.06 | 98 |
NSDC 3 | 49.944 | 0.330 | 50.27 | 101 |
NSDC 4 | 49.125 | 0.431 | 49.56 | 99 |
NSDC 5 | 47.781 | 0.453 | 48.23 | 96 |
NSDC 6 | 49.679 | 0.458 | 50.14 | 100 |
NSDC 7 | 49.726 | 1.168 | 50.89 | 102 |
NSDC 8 | 43.359 | 0.312 | 43.67 | 87 |
NSDC 9 | 49.320 | 2.597 | 51.92 | 104 |
NSDC 10 | 49.044 | 0.535 | 49.58 | 99 |
Mean (±SD) | 48.65 (±2) | 0.7 (±0.7) | 49.35 (±2.2) | 99 (±4) |
Table 1: Dose uniformity testing for a beclomethasone dipropionate nasal spray with automated actuation (Vertus II) and NSDC for dose collection.
Copley’s NSDC has a curved impaction surface that minimises dripping onto the spray nozzle tip and an internal geometry that guides the collected sample to a small collection sump. The Vertus II interfaces directly with the NSDC, fully automating actuation and test flow control. The speed, angle and duration of shaking ahead of actuation; firing force, including the speed of application and release of that force; and time delay between shaking and firing can all be defined and closely controlled, in accordance with test requirements.
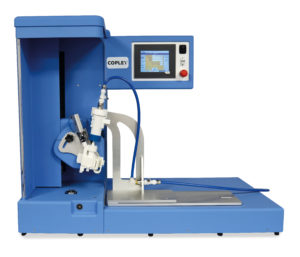
Figure 1: A test set-up for dose uniformity testing with automated actuation (Vertus II) and MDI DUSA.
In the single actuation content study, testing was carried out using a commercial beclomethasone dipropionate formulation filled into a standard commercial nasal spray pump/bottle (Aptar Pharma). The delivered dose was determined by opening the NSDC and recovering the drug via a process of rinsing and sonication. Drug was also recovered from the nasal spray nozzle via rinsing and dissolution. The total metered dose was determined by summing the drug recovered via both routes and then compared with the label claim. The results showed extremely high repeatability across a series of ten tests, far higher than the 75–125% acceptability limits specified in the European Pharmacopoeia.9 As discussed, such precision translates into high sensitivity, enabling studies to differentiate between products more accurately.
Figure 1 shows an alternative test set-up for automated dose delivery measurement using a standard metered dose inhaler (MDI) dose uniformity sampling apparatus (DUSA). Recent revisions to the European Pharmacopoeia and USP <601> updated guidance on delivered dose uniformity for nasal sprays, aligning it with guidance for pressurised MDIs.8,9 Direct interfacing of the DUSA with the Vertus II provides a simple, easy-to-use and high integrity set-up for automated testing.
A BENCHTOP TOOL FOR ASSESSING REGIONAL DEPOSITION
Recognition of the potential benefit of a better benchtop tool for investigating regional deposition for nasal drug products has led to the development of the Alberta Idealised Nasal Inlet (AINI),10 using analogous methodologies to those deployed in the development of the Alberta Idealised Throats. Both products were originally developed by researchers working with Professor Warren Finlay at the University of Alberta (Edmonton, Canada) and subsequently commercialised by Copley Scientific. Rather than realistic replicas of the nasal cavity or throat, these “idealised” accessories are easy-to-use tools that accurately mimic deposition behaviour for a wide range of patient physiologies.
Development work for the AINI began with computational fluid dynamic simulations of drug deposition behaviour in realistic nasal airway geometries derived from computer tomography (CT) scans of seven adult subjects.7 Close to 250,000 data sets were produced to rigorously assess the impact of various parameters, including droplet size and injection velocity, for sprays injected separately in both left and right nostrils. An idealised geometry was developed from these data,6,9 striking a balance between an accurate representation of the deposition behaviour observed in the replicas and a simple, easy-to-use solution (Figure 2).
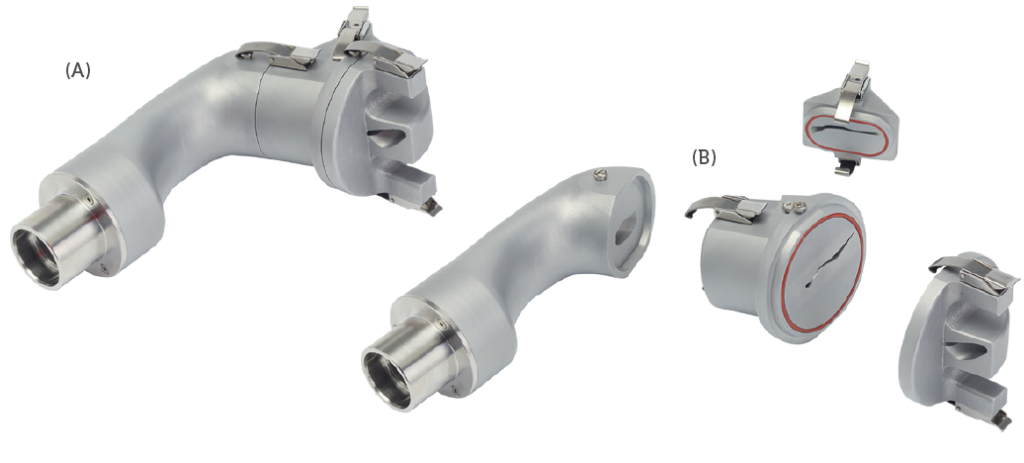
Figure 2: An image of the AINI (A) split to allow study of the four regions of interest (B)
Figure 3 shows data from an experimental study of the performance of the idealised geometry relative to realistic nasal replicas.11,12 In this work, five polymeric nasal replicas were manufactured from CT data gathered from adult subjects, using a rapid prototyping technique. These were then used to assess drug deposition from a commercial nasal spray pump (specifically a 5.2 mg cromolyn sodium spray). Testing was carried out at an inspiratory flow rate of 7.5 L/min at two different orientations: 60° and 45° from horizontal. Comparative testing was also carried out with a rendering of the idealised geometry produced using the same prototyping technique. Note that, in this study, the element of the idealised geometry referred to as “vestibule” in the AINI is referred to as “vestibule + valve” for comparison with the nasal replicas.
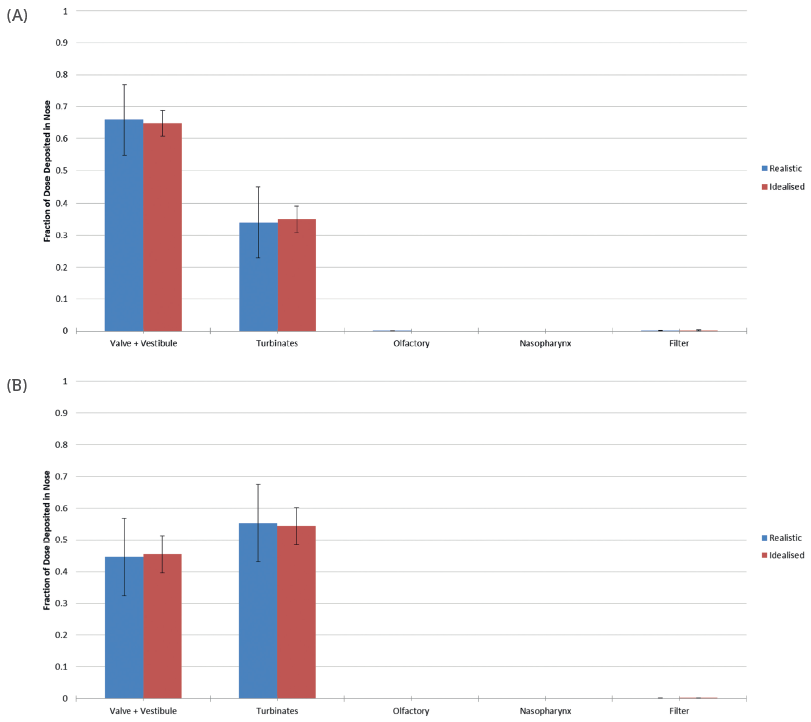
Figure 3: Deposition data for a commercial nasal spray, at an orientation angle of 60° (A) and 45° (B), highlight the ability of the idealised geometry to accurately reflect behaviour in realistic nasal geometries and reduce variability.
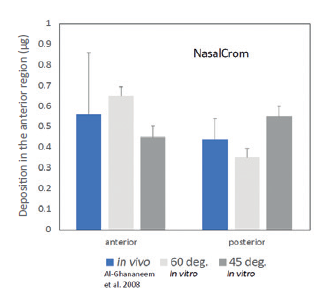
Figure 4: Deposition data for a commercial nasal spray measured with the idealised geometry show good agreement with in vivo gamma scintigraphy data.
The results showed close agreement between the data generated using the idealised geometry and the realistic nasal replicas. However, the results generated with the idealised geometry exhibited far less variability, enhancing their value for scientific studies. Interestingly, the data gathered at different orientations showed a significant difference in deposition behaviour, underlining the importance of this patient technique-related variable in the drug delivery process.
For this nasal spray there are also published gamma scintigraphy data quantifying in vivo deposition in five male subjects.4 In this work, average deposition in the anterior region, which translates approximately to the valve + vestibule, was found to be 0.56 ± 0.3 μg, while posterior deposition, which can be equated with deposition in the turbinates, was 0.44 ± 0.1 μg. The administration angle was not reported in this work, but this compares with 0.45–0.65 μg and 0.35–0.55 μg respectively, depending on orientation (Figure 4). In both studies deposition in the nasopharynx was found to be negligible.
These results confirm the relevance and utility of the AINI which, in practice, has two key applications. Firstly, it can be attached directly to an external filter to directly investigate regional deposition, as exemplified by this study, as well as to representatively measure respirable mass – the mass of dose that may reach the lung. Such studies are valuable for targeting drug delivery in new drug development and for the demonstration of bioequivalence in generic product development. The second application is to interface the AINI with a cascade impactor to representatively determine the amount of drug in small particles/droplets with a higher degree of clinical realism. An inlet that fails to simulate drug capture in the nasal cavity prevents representative assessment of the respirable dose.
GOING FORWARD
Better solutions for in vitro testing have an important role to play in accelerating the development of nasal drug products, whether the goal is new vaccines for covid-19, more clinically efficacious therapies for neurological diseases or cost-effective products for everyday ailments that negatively impact quality of life. Automation can reduce the variability associated with testing, sharpening sensitivity while also boosting analytical productivity. On the other hand, benchtop tools for the study of regional deposition are a cost-effective option for researchers investigating how to target specific areas of the nasal cavity to enhance drug delivery, as well as for those looking to support claims of bioequivalence. For new and generic nasal product developers alike, these tools are an important step forwards in efforts to exploit the full potential of the nasal route for drug delivery, and realise the exciting possibilities it promises.
REFERENCES
- Mittal D et al, “Insights Into Direct Nose to Brain Delivery: Current Status and Future Perspective”. Drug Deliv, Mar 2014, Vol 21(2), pp 75–86.
- Ramvikas M et al, “Nasal Vaccine Delivery” in “Micro and Nanotechnology in Vaccine Development” (Skwarczynski M, Toth I, eds). Elsevier, 2017, pp 279–301.
- “University of Oxford to Study Nasal Administration of COVID-19 Vaccine”. Press release, University of Oxford, Mar 2021.
- Al-Ghananeem AM et al, “Gamma Scintigraphy for Testing Bioequivalence: A Case Study On Two Cromolyn Sodium Nasal Spray Preparations”. Int J Pharm, 2008, Vol 357(1–2), pp 70–76.
- “Draft Guidance for Industry: Bioavailability and Bioequivalence Studies for Nasal Aerosols and Nasal Sprays for Local Action”. US FDA, Apr 2003.
- “FDA Approves First Treatment for Severe Hypoglycemia That Can Be Administered Without an Injection”. Press release, US FDA, Jul 2019.
- Kiaee M, “Regional Deposition of Nasal Sprays in Adults: A Wide Ranging Computational Study”. Int J Numer Method Biomed Eng, May 2018, Vol 34(5), Article 2968
- “USP<601> Aerosols, Nasal Sprays, Metered Dose Inhalers and Dry Powder Inhalers”. US Pharmacopeial Convention, 2020.
- “Nasal Preparations”. European Pharmacopoeia, Chapter 10.5.10. Kiaee M, “An Idealized Geometry that Mimics Average Nasal Spray Deposition in Adults: A Computational Study”. Comput Biol Med, 2019, Vol 107, pp 206–217.
- Kiaee M, “An Idealized Geometry that Mimics Average Nasal Spray Deposition in Adults: A Computational Study”. Comput Biol Med, 2019, Vol 107, pp 206–217.
- Finlay WH et al, “Development of an Idealized Nasal Geometry for Estimation of Regional Deposition of Nasal Sprays”. RDD 2021, 2021, Vol 1, pp 127–134.
- Chen JZ et al, “In Vitro Assessment of an Idealized Nose for Nasal Spray Testing: Comparison with Regional Deposition in Realistic Nasal Replicas”. Int J Pharm, 2020, Vol 582, Article 119341.