To Issue 148
Citation: Cape J, “Modified-Release Microspheres: Maximising Coating Integrity and Optimising Release.” ONdrugDelivery, Issue 148 (May/Jun 2023), pp 8–11.
Jonathan Cape discusses development considerations and workflows to select optimal enteric coat weights for modified release microspheres – balancing performance considerations with API stability and the excipient burden to patients.
Modified-release (MR) microspheres are widely used intermediates for dosing compounds that require protection from the gastric environment. They can also deliver drugs to the site of action in the upper and middle gastrointestinal tract, such as the duodenum or jejunum. Polymeric enteric coatings are often used on top of an immediate-release or MR inner core particle to give MR microspheres this type of additional functional performance.
“Upper limits to certain excipients may also be dictated by the acceptable daily intake of the target patient population.”
Manufacturing technologies to make these microspheres are well established. For making the inner drug-containing cores, these include spray layering, melt-congeal and granulation methods. Fluid bed techniques are commonly used to apply the enteric coatings. However, developing new enteric- coated microspheres requires the correct selection of polymeric coating, plasticisers and anti-tacking agents, along with an appropriate inner core matrix material. Moreover, the weight of coating applied affects both manufacturability and enteric functionality, and these need to be balanced. Other excipient considerations include minimising the impacts on API stability in the drug product formulation and level of impurities in the excipients.
Managing performance versus excipient burden considerations can be a primary driver for formulation decisions, as MR microspheres often require heavy coat weights to achieve strictly enteric functionality. It can be above 50% in some cases, representing a significant amount of the excipient burden to a patient. This burden can be critical for vulnerable patient populations, such as paediatrics.
COATING PROCESSES
Fluid bed coating is the most commonly used unit operation to apply enteric coatings to small microsphere intermediates with diameters ranging from about 0.1 to 0.5 mm. This unit operation consists of partially entraining particles in a vertically directed airflow from a fluidised bed charged with microspheres through a central vertical column (a Wurster column).
In the bottom spray configuration, a spray nozzle located within the Wurster column coats the particles as they pass through the spray plume emerging from the nozzle. Particles de-entrain and fall back to the fluidised bed and are then repeatedly passed through the column, gradually building up the coat on the uncoated particles. The batch will finally reach an endpoint based on a target that has been set for the average applied coat weight.
Excipient impacts on API stability and patient toxicity are generally assessed early on in a programme. This allows the formulation approach to be aligned with the quality target product profile. Both stability and toxicity determine the maximum tolerable coat weight in a formulation, providing upper limits to the amounts of impurities that may be present in the excipients used to coat. For example, enteric polymers may contain trace impurities such as peroxides, aldehydes and free acids that can impact API stability. Minimising the source of these impurities through coat weight control is one way in which the stability of the drug product can be ensured.
Upper limits to certain excipients may also be dictated by the acceptable daily intake of the target patient population. This applies to enteric coatings and additives within them, including plasticisers and anti-tacking agents. As an example, toxicology data is often lacking in paediatric populations for novel coating formulations, and therefore upper dosing limits are often inferred from animal studies or demonstrated safety in adult formulations. One general strategy is to maintain excipient doses well below demonstrated safe levels for adults when used in indications for sensitive populations.
Formulators must also ensure that the coating itself performs its intended function and achieves the target specifications for enteric dissolution. Two main factors are relevant when selecting the target applied coat weight for the enteric coating. First, enteric performance is generally only achieved if a sufficiently high threshold average coat thickness is applied to the particles. This threshold varies considerably between coating formulations and is often empirically determined by making a performance assessment of intermediate coat weight samples from an initial fluid bed survey run. Sufficient coat thickness ensures that, on average, the coating completely covers the texture of the underlying core particle, and that coating defects are buried under additional coating material to achieve optimal integrity of the coat.
Second, the average applied coat weight needs to be sufficiently high so that it accounts for the coat weight variations that will inevitably occur across the range of the core particles’ size distribution. In other words, the applied coat weight is generally not equal for all sizes of core particle; this variation is referred to as the coat weight distribution. This coating heterogeneity can have critical implications for early burst release in coated microsphere formulations.
“A sharp transition in early-release behaviour will often be observed as the coat weight rises above a critical level.”
COAT WEIGHT DISTRIBUTION
To understand how coat weight distributions arise, consider a general case where particles traversing the spray plume each have an equal probability of being hit by coating droplets. These particles may be prone to pick up equal coat thicknesses across the core population. This situation is commonly encountered in fluid bed coating and will result in larger particles having a lower relative coat weight than smaller particles. These larger, less-coated particles may exhibit early release if an osmotic potential exists in the core particle.
Alternatively, under other fluid bed coating conditions, particles may accumulate coating in a manner proportional to their cross-sectional area. In this case, each particle within the core population may pick up an equivalent coat weight, but the coat thickness may be vastly different between large and small particles on account of the nature of the surface area to volume. This limiting case leads to the opposite behaviour. Here, small particles will have thinner coatings, and larger particles will have thicker coatings. Both these limiting case examples implicate insufficient coat weight or coat thickness as root causes of early release in enteric-coated microsphere populations. This is illustrated in Figure 1.
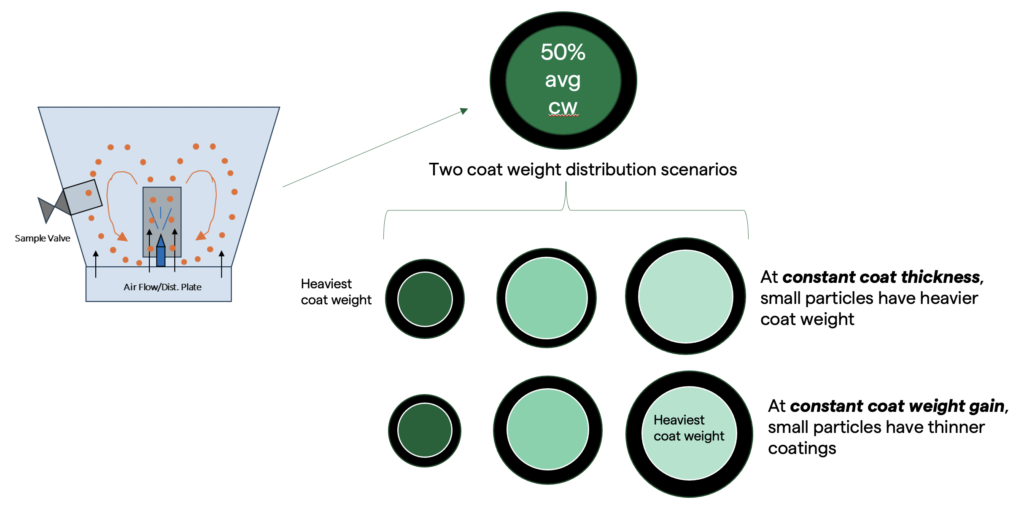
Figure 2: Workflow for enteric coat optimisation.
It is worth considering the overall workflow for enteric coat optimisation, as shown in Figure 2. Fortunately, several tools in development workflows are available that allow formulators to pinpoint the threshold for sufficient coat weight in prototype formulations, and then understand dissolution failure modes and sensitivity in terms of the formulation and process space. Drawing intermediate coat weight samples during an initial prototype coating run is commonly performed to test the sensitivity of overall release to the average coat weight.
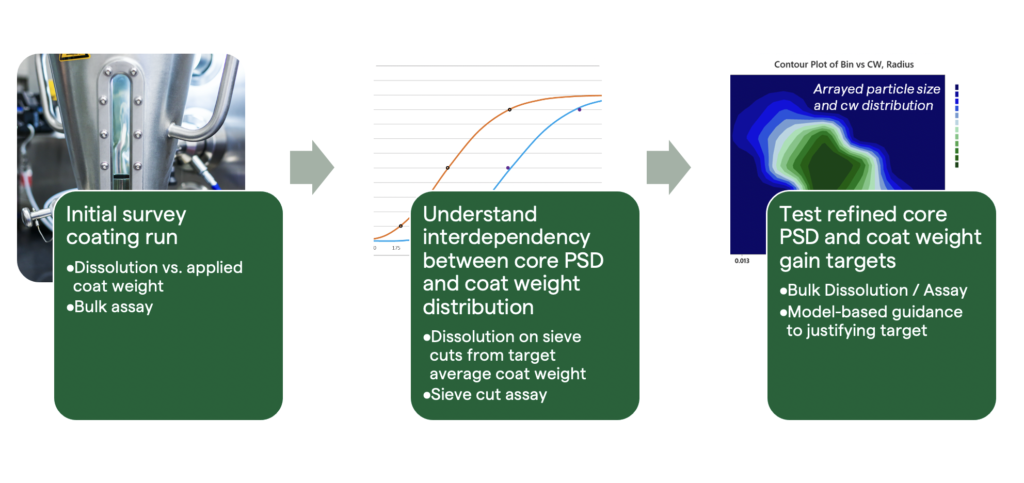
Figure 2: Workflow for enteric coat optimisation.
This experiment is essential to determine the initial ballpark target for the coat weight required to achieve the enteric-release target. A sharp transition in early-release behaviour will often be observed as the coat weight rises above a critical level, which may vary with core and coating formulations. Early release may still be observed, even at coat weights approaching or exceeding the upper limits established by the stability and toxicology considerations. If this occurs, a deeper dive is required to gain a better understanding of the nature of early release and then explore mitigation strategies.
Further experimentation may involve separating the target coat weight population by sieving it into sub-populations with different particle sizes. If the enteric dissolution performance of these sub-populations is measured, it should reveal the mechanism driving early release.
“If increasing assay is observed with increasing overall particle size, then the coat weight distribution is consistent with a constant coat thickness model.”
It should also make it possible to determine whether the fluid bed coating process is taking place on a constant coat thickness or a constant coat weight basis. For example, if increasing assay is observed with increasing overall particle size, then the coat weight distribution is consistent with a constant coat thickness model. In this case, the larger particles will be under-coated relative to the average target coat weight established using bulk measurements, making this part of the population more prone to either passive permeation or osmotic burst mechanisms.
PASSIVE PERMEATION OR OSMOTIC BURST?
Simulation and further experimentation can distinguish between passive permeation and osmotic burst mechanisms, which, in turn, can lead formulators to a viable control strategy for dissolution performance. Simple micrographic evidence is often sufficient to demonstrate an osmotic burst mechanism, in which particles can be seen to swell and then burst after a lag phase. This behaviour is observed only in a subset of particles, thus providing strong evidence for the under-coating of a specific sub-population of particles within the core population. Further, this data can be quantitatively modelled according to osmotic flux and passive permeation models for early release. One such model is shown in Figure 3.
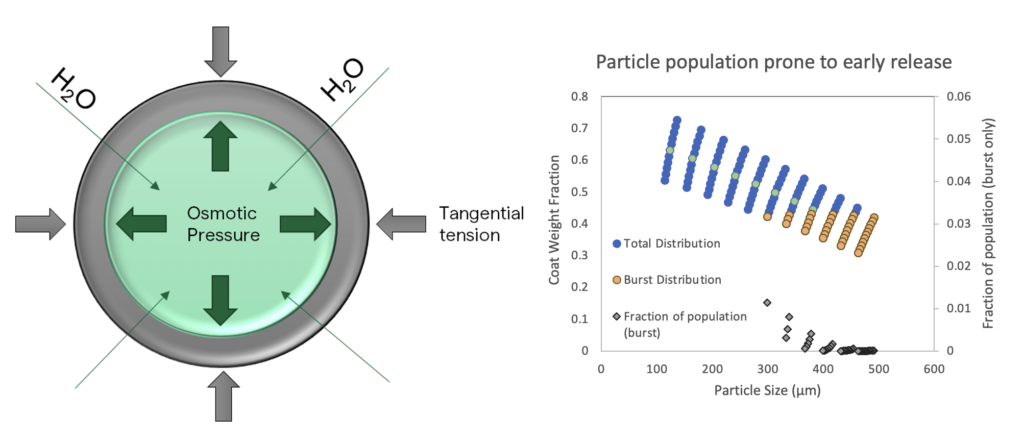
Figure 3: Predictive model of coat weight distribution and dissolution impact.
“If the inner osmotic potential exceeds the outer coating’s ability to contain it, the particle will burst.”
Many core particle formulations incorporate water-soluble components. These include the drug itself, of course, but also excipients such as functional polymers, binders and fillers. While specific formulations are beyond the scope of this discussion, microsphere core formulations will nearly always have some propensity for water uptake.
Polymeric enteric coatings have a propensity for water uptake, too, making them permeable upon soaking, and allowing a limited influx of water or media into the inner core particle. This influx is governed by the osmotic potential of the core, which can be modelled with equations of state or mixing functions, such as the Flory– Huggins solution theory. The inner core necessarily swells, but the coating around it tends to resist swelling. When the osmotic force within the particle and the effective modulus of the outer coating are equal, the particle is in mechanical equilibrium and will maintain its swollen size. However, if the inner osmotic potential exceeds the outer coating’s ability to contain it, the particle will burst. This mechanism appears to explain early burst release in many cases.
Passive permeation differs, as it will not result in particle swelling, and neither will early bursting be observed in micrographic soak test studies. This mechanism of early release is strongly impacted by both the coating formulation and coat weight. As with osmotic bursting particles, the passive permeation mechanism is also subject to coat weight distribution impacts. Particles with thinner coatings will exhibit reduced lag times to early release, and faster permeation once release begins. However, in contrast to the osmotic burst mechanism, early release resulting from passive permeation can be expected to occur throughout the entire particle population.
MITIGATION STRATEGIES
Once these mechanisms have been identified and understood, mitigation strategies may be developed around the formulation and coating process. For example, if a thinly coated sub-population with a high particle size is found to be responsible for early release, a control strategy around ingoing core particle size may be implemented to mitigate it. Modifications to the average target coat weight may also be employed to bump the formulation into a better performance space.
Alternatively, strengthening the coating by incorporating high-molecular-weight polymers may enable the formulation to resist osmotic swelling. Passive permeation may be mitigated by similar approaches: targeting and eliminating thinly coated sub-populations, modifying the overall coat weight target and altering the coating’s formulation. Reducing the levels of, or even eliminating, plasticisers or water soluble additives in the formulation may also help.
Designing enteric-coated microspheres can be a nuanced process, but this can be made easier if target product profile constraints are carefully considered at the outset and appropriate workflow tools are in place. Establishing upper limits to coat weight based on API stability and patient excipient burden considerations should be the first step in designing an enteric-coated microsphere product design.
Using simple experimental tools alongside modelling can help to establish acceptable performance based on target coat weight, whereas fine-tuning the coat weight distribution allows performance, stability and other aspects of the target product profile to be optimised. These workflows allow the empiricism to be taken out of enteric-coating optimisation, leading to robust and scalable products.