To Issue 145
Citation: Baron C, Ganley W, “Navigating pMDI Reformulation 2.0”. ONdrugDelivery, Issue 145 (Apr 2023), pp 12–17.
Chris Baron and William Ganley examine possible responses to the environmental impact of pressurised metered dose inhalers use and consider alternative inhaler types, with a focus on the strategies and support that can accelerate the development of the next generation of pMDIs.
“Ensuring continuity of treatment and patient safety is critical as we
work towards more sustainable pMDIs.”
In 2019, it was estimated that asthma affected over 250 million people worldwide, causing 455,000 deaths.1 Such statistics put into context the criticality of pressurised metered dose inhalers (pMDIs), our cost-effective, frontline defence for common respiratory disease. Estimates for 2020 indicate that close to ~70% of portable inhaler sales (by volume) were pMDIs, and that pMDIs accounted for >95% of rescue medications.2 Even as we recognise our responsibility to minimise the environmental impact of pMDI use, we must acknowledge their value in preserving life and quality of life. Ensuring continuity of treatment and patient safety is critical as we work towards more sustainable pMDIs.
Concerns over the environmental impact of pMDIs are not new. The signing of the Montreal Protocol in 1987 triggered industry-wide reformulation to phase out chlorofluorocarbons (CFCs), ushering in the use of more environmentally benign hydrofluorocarbon (HFC) propellants. Now, we have aggressive and challenging targets to phase down the use of high global warming potential (GWP) products and the reformulation cycle is underway once more. Key players such as Chiesi, AstraZeneca and GSK have announced ambitious plans to reduce the carbon footprint of their pMDI products by upwards of 90% in the next few years by switching to alternative low-GWP propellants, which have a much lower carbon footprint.
This article examines possible responses to the environmental impact of pMDI use and considers the pros and cons of alternative inhaler types and lessons to be drawn from earlier reformulation activities. A key focus is on the strategies and support that can accelerate the development of the next generation of pMDIs, including the end-to-end services available from Aptar Pharma.
ARE pMDIS THE ONLY ANSWER?
Given the availability of alternative inhaler platforms, it is reasonable to ask whether simply reducing our reliance on pMDIs would be the best way forward.
A switch to dry powder inhalers (DPIs) is an obvious potential solution since this is well-established propellant-free technology with a correspondingly lower CO2 footprint, however, the net CO2 footprint will be dependent on the design/type of DPI and the complicities of the respective manufacturing processes. DPIs undoubtedly have some compelling attractions. They enable the delivery of larger drug loadings and capsule devices, in particular, are relatively inexpensive.
For pharmaceutical companies, both multidose and reservoir DPIs provide considerable opportunity for establishing high-value intellectual property (IP) but the corollary for healthcare providers is high cost and a multiplicity of device designs. Consequently, interchangeability and patient experience can be a major concern with respect to patient adherence and compliance. Switching a patient from a pMDI to any DPI requires care, training and monitoring to ensure ongoing, effective health management and, crucially, DPIs are not suitable for all patients or for rescue medication. Patients with compromised or reduced inspiratory effort may not have sufficient inspiratory flow rate to trigger the DPI, compromising dose delivery.
Soft mist inhalers are an alternative. These use propellant-free technology to generate a mist of fine droplets of consistent size and have a low CO2 footprint relative to pMDIs. Patient experience is more akin to a pMDI, but cost is an issue and the technology is restricted to solution formulations, limiting application to drugs with appropriate solubility.
In summary, while we may be able to increase our use of alternative devices, notably for health maintenance, reformulating pMDIs with more sustainable propellants is vital, particularly for low- to middle-income countries and for the trusted delivery of rescue medications. Success will deliver safe therapeutics with an acceptable CO2 footprint in a design familiar to patients, at accessible cost.
“Patient adherence and compliance could be further improved through the increased adoption of, for example, dose counters, breath-actuated inhalers and connectivity.”
MEET THE NEW PROPELLANTS
HFA 152a and HFO 1234ze are currently the leading low-GWP propellant candidates for pMDI reformulation. Table 1 summarises their key properties.
Turning first to HFA 152a (1,1 difluoroethane), this has a GWP >90% less than current propellants and has come up clean in a two-year toxicology programme (Lovelace Biomedical, sponsored by Koura , MA, US). A US FDA Drug Master File (DMF) was scheduled to be in place by the end of 2022, facilitating its use for reformulation activities. Flammability is a safety concern, primarily for manufacturing, but issues are manageable, albeit at additional cost.
Propellant | Formula | B. Pt ˚C | Density @20˚C g/mL |
Ozone depleting potential | Global warming potential (CO2 = 1) |
CFC 11 | CFCI3 | 23.7 | 1.49 | 1 | 4,660 |
CFC 12 | CF2CI2 | -29.8 | 1.33 | 1 | 10,800 |
HFA 134a | CF3-CFH2 | -26.2 | 1.23 | 0 | 1,300 |
HFA 227a | CF3-CFH2 | -16.5 | 1.41 | 0 | 3,350 |
HFA 152a | CF2H-CH3 | -24.7 | 0.91 | 0 | 138N |
HFO1234ze | CF3CH=CHF3 | -18.9 | 1.29 | 0 | <1 |
Table 1: Key properties of original CFC pMDI propellants, HFC replacements and next-generation low-GWP candidates.
HFO 1234ze (1,3,3,3 tetrafluoroprop-1-ene), the alternative candidate, has an even lower GWP and an associated potential to reduce the CO2 footprint by ~99%. A DMF has already been submitted to the FDA, allowing companies to file IND applications, and GMP-grade HFO 1234ze is now available for testing and in commercial quantities. Toxicology data has been reviewed by the FDA, and Phase III studies are planned (Honeywell).
These two propellants are promising candidates for substitution. So, are there lessons to be drawn from the transition from CFCs to HFCs to accelerate progress towards their commercial use?
WHAT CAN HISTORY TEACH US?
There is a balance to be struck when reformulating between a “copy and paste”, minimally disruptive approach, and taking the opportunity for significant product improvement. If we can establish a clear definition of the target product profile, then there is potential to simultaneously enhance other aspects of device and formulation performance. For example, in the last transition we learned that the inclusion of ethanol enabled smaller metering volumes. Duplicating this effect in the current phase down could magnify the CO2 footprint reduction associated with propellant substitution. Switching to, for example, primeless valves would similarly augment environmental gains and, at the same time, ease patient use. Patient adherence and compliance could be further improved through the increased adoption of, for example, dose counters, breath-actuated inhalers and connectivity.
The shift to HFCs brought considerable challenges with respect to formulation stability and IP linked to the use of specific surfactants. In this respect, HFA 152a and HFO 1234ze appear to present a lesser challenge with behaviour somewhat similar to the currently used HFCs. Key formulation decisions to take this time centre on:
- Whether to develop a suspension or solution
- The extent of ethanol inclusion
- The impact of any inactive ingredients included.
Addressing the flammability of HFA 152a is also a focus as attention moves to filling/manufacturing, but propellant suppliers are more involved in the reformulation process this time around, which may be particularly helpful when it comes to tackling this issue.
TRACKING THE IMPACT OF REFORMULATION FROM ACTUATION TO EFFICACY
Against this background of clear motivation and targets, and armed with learnings from the past, we can look in more detail at the reformulation process. Despite considerable advances in our understanding of pMDIs over the last 20 years, the challenge remains significant.
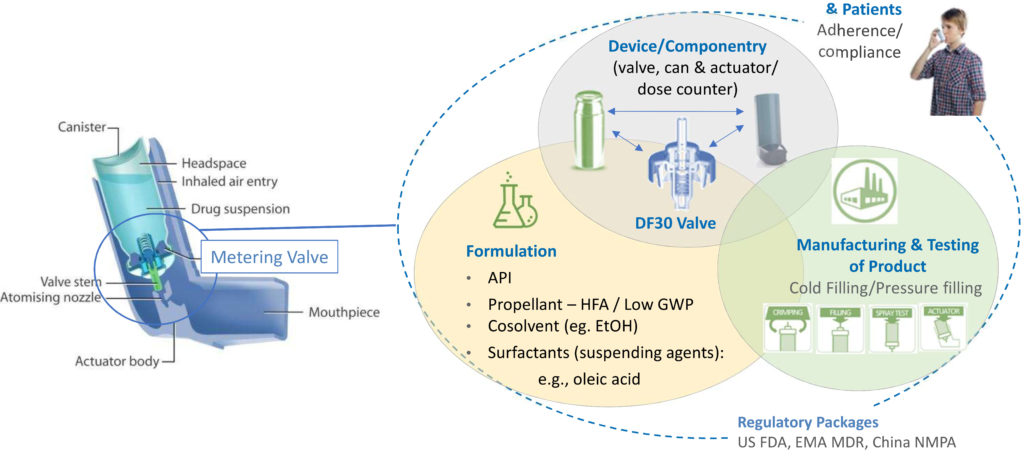
Figure 1: Reformulation involves rigorous and systematic consideration of a network of interacting factors centred on the formulation, the device and the manufacturing process.
Figure 1 shows the network of interrelated issues associated with development, use and commercialisation of a pMDI. The metering valve is rightly a primary focus, necessarily optimised in combination with the evolving formulation, taking into account all associated componentry and the intended manufacturing process. For instance, will the metering chamber volume need to be fine-tuned to deliver an equivalent dose to the existing pMDI product? Are there interactions between the proposed formulation and the closure containment system and actuator that necessitate a change of material or alternative approach? Is the valve compatible with a filling and crimping process that is appropriate for the new formulation? Wrapped around the central optimisation challenge is the need to develop a robust regulatory package and to recognise the influence of patient practice and technique.
“Solubility of the active drug substance directs towards formulation as either a solution or suspension.”
Optimally leveraging in vitro and in silico methods to access the information required to progress is essential and can be difficult. This is where SmartTrack™ can help. SmartTrack is a toolkit that draws together Aptar’s collective expertise to accelerate pMDI reformulation projects. In vitro tests are packaged in Smart Track, which generates clinically relevant data, with in silico models that simulate lung deposition and pharmacokinetics (PK). Using the results, key activities can be accelerated, such as dose-ranging studies and excipient selection and, ultimately, minimise the number and size of clinical trials required to support commercialisation.
Tools and techniques earn their place in a pMDI reformulation toolkit by providing information that promotes faster, more secure progression. The following are used with SmartTrack:
- Particle image velocimetry and phase Doppler anemometry (PDA) to quantify plume dynamics and elucidate interactions between the device, inhalation profile, co-ordination and oropharynx that define behaviour in the initial stages of drug delivery
- Aerosol testing with anatomically realistic mouth-throat models and representative breathing profiles to study the deposition of drug particles
- Algebraic and computational fluid dynamic models to model the deposition of drug particle transport and deposition in the airways
- Microstructural characterisation and combined dissolution/permeability studies to investigate release of the active drug substance, post-deposition
- Validated physiologically-based PK (PBPK) models to predict clinical outcomes for specific patient populations from in vitro inputs
- Accelerated stability testing and extractable/leachable profiles to detect and mitigate stability and device component compatibility issues as early as possible.
Let’s take a closer look at some of the data already generated using these, and other complementary tools, to gain insight into the physicochemical properties of lower GWP propellants and support reformulation activities.
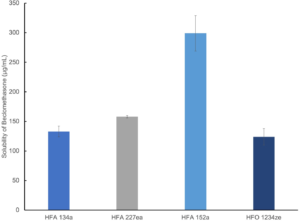
Figure 2: Beclomethasone is markedly more soluble in HFA 152a than in alternative propellants.
Study 1: Exploring Drug Solubility
Figure 2 shows solubility data for beclomethasone in current and next-generation propellants measured using high-pressure nuclear magnetic resonance.
Solubility of the active drug substance directs towards formulation as either a solution or suspension. For solution formulation, solubility influences the need for co-solvent while, in a suspension formulation, it controls particle size stability. Here, beclomethasone was found to have substantially higher solubility in HFA 152a (400 µg/mL) than in the other three propellants – signposting an opportunity for solution-based formulation with relatively low levels of co-solvent.
Study 2: Investigating Droplet Dynamics
Figure 3 shows droplet size and velocity data for four placebo propellant-only formulations measured as a function of distance from the mouthpiece, using PDA, at a test flow rate of 30 L/min. Data were also measured under no-flow conditions (not shown).
Droplet size and velocity impact aerosol evaporation rate in the earliest stages of drug delivery, thereby affecting drug deposition in the airways. Under no-flow conditions (0 L/min) plume dynamics were closely comparable with all propellants but at 30 L/min (the rate specified for compendial testing for pMDIs) there are clear differences. Lower-GWP propellants result in larger droplets close to the mouthpiece, although this trend does not persist; larger droplet sizes are recorded at greater distances with HFA 227 and HFO 1234ze. In summary, a somewhat complex and differentiated pattern in droplet size evolution emerges, which may be helpful in elucidating deposition in the mouth and throat and determining what reaches the lung.
Plume velocity trends are simpler with velocity decaying with all propellants as a function of distance from the mouthpiece; HFA 152a exhibits the slowest decay, HFA 227 the fastest.
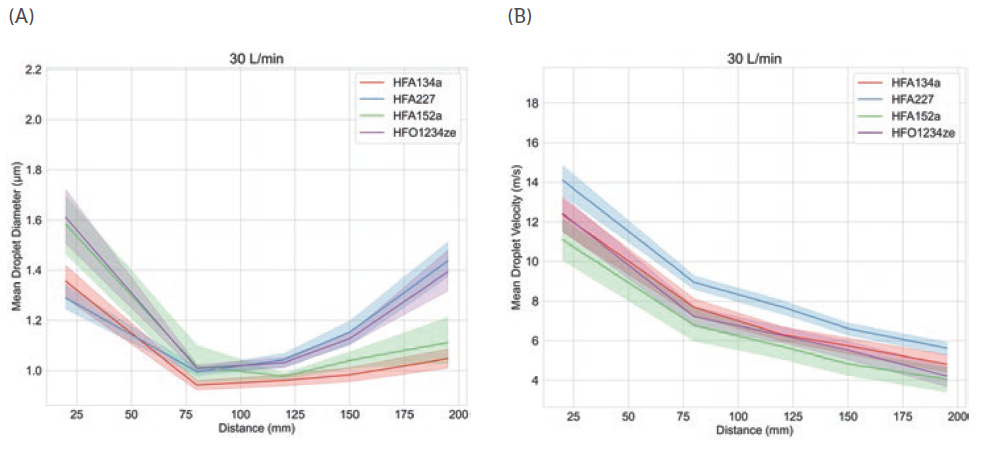
Figure 3: Propellant choice directly influences plume dynamics notably with respect to changes in droplet size as a function of distance from the mouthpiece (left).
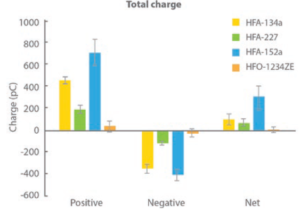
Figure 4: All propellants produce droplets carrying a net positive charge, but low-GWP propellants are highly differentiated; HFA 152a is associated with a high net charge while HFO 1234ze results in particles with minimal charge.
Study 3: Investigating Net Charge
Figure 4 shows charge data for droplets produced with each of the propellants, as a function of droplet size, measured in collaboration with the University of Sydney (Australia) using a bipolar charge analyser.
During propellant evaporation, droplets may acquire charge, which can influence deposition behaviour, notably in the deep lung. In this experiment, all propellants were found to produce a net positive charge on finer particles, but the magnitude of that charge varied significantly. HFA 152a resulted in the highest net charge with HFO 1234ze, in contrast, resulting in a net charge close to zero.
Further measurements identified a direct correlation between the water content of the propellant and net charge. A corresponding hypothesis is that the rapid temperature drop associated with propellant evaporation causes the formation of ice crystals that carry a charge, potentially the charge measured by the test. It is worth noting that water content is a function of the as-supplied propellants, so while these results may not indicate that the propellant droplets themselves are charged, they undoubtedly have implications for formulation.
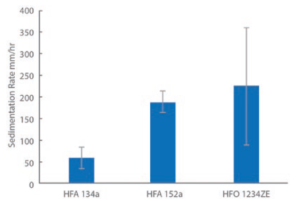
Figure 5: Sedimentation rates for albuterol sulfate are relatively high in low-GWP propellants.
Study 4: Exploring Sedimentation Rate
Figure 5 shows sedimentation data for HFA 134a and the two lower-GWP propellants measured for albuterol sulfate formulations, analogues of a commercial albuterol sulfate formulation (Ventolin, GSK) made with alternative propellants. These formulations were not optimised – the propellants were simply switched out to assess their differences.
Study 5: Assessing Drug Deposition
Figure 6/Table 2 shows drug deposition data for the commercial and analogue albuterol sulfate formulations. Cascade impaction was carried out using a realistic mouth-throat interface to determine aerodynamic particle size distribution (APSD) metrics, which were then used in an algebraic lung deposition model to compare likely drug deposition profiles.
Formulation | ED (μg) | BB (0-8) (μg) | Bb (9-15) (μg) | AL (16-23) (μg) | EX (μg) |
Ventolin | 77.89 | 1.22 | 2.33 | 26.67 | 3.00 |
Albuterol HFA-152a |
78.04 | 1.37 | 1.58 | 22.01 | 2.32 |
Albuterol HFO-1234ze |
98.52 | 1.51 | 2.65 | 17.22 | 1.03 |
Figure 6/Table 2: Regional Deposition Modelling using 1-D Lung Models.
“Further measurements identified a direct correlation between the water content of the propellant and net charge.”
These tests provide insight into how the observed physicochemical differences manifest in drug delivery performance. Relative to Ventolin, the HFA 152a formulation delivers a lower emitted dose (ED), potentially because of its lower density, but a comparable fine particle fraction (FPF). With HFO 1234ze, ED is closer to that of the reference product, but FPF is substantially lower. Plume dynamics and flocculation both provide a possible rationale (Table 3).
Formulation | ED/μg | ISM/μg | MMAD/μg | GSD | FPM/μg <5μm |
FPF/% |
Ventolin | 111.12 | 72.04 | 2.22 | 2.26 | 54.27 | 58.68 |
Albuterol HFA-152a |
105.32 | 65.79 | 2.13 | 2.50 | 50.18 | 58.70 |
Albuterol HFO-1234ze |
108.91 | 51.00 | 2.29 | 2.60 | 38.32 | 34.89 |
Table 3: APSD metrics and deposition modelling highlight the poor performance of the unoptimised HFO 1234ze formulation relative to the commercial analogue; HFA 152a produces drug delivery performance intermediate between the two.
The modelling results illustrate the implications of these metrics for drug deposition. Both low-GWP propellants result in markedly reduced drug delivery to the deep lung, the outer, darker coloured region in each image, with the HFO 1234ze formulation substantially increasing the extra-thoracic dose, the dose depositing in the mouth and throat (see Figure 6).
THE WAY FORWARD
Our reliance on pMDIs, particularly for emergency treatment, makes them essential. Reformulation to deliver a lighter CO2 footprint is therefore critical within the constraint of safeguarding patient health. Collectively we have tackled extensive pMDI reformulation before but, this time around, we have more knowledge to guide us and better tools to use. Aptar Pharma is already using its skills to characterise the most promising low-GWP propellants for reformulation and develop technology to deliver their potential. By providing customers with cutting-edge expertise and tools to accelerate and improve every step of the reformulation journey, the company is reducing the work and risk involved in commercialising the next generation of pMDIs.
For more information about sustainable pMDIs, visit: https://www.aptar.com/resources/investigating-the-propellant-pathways-leading-to-a-sustainable-future-of-mdis.
REFERENCES
- “Asthma”. WHO Fact Sheet. May, 11 2022.
- Internal survey data, Aptar.