To Issue 142
Citation: Zeiss B, “Silicone-Oil-Free Prefilled Syringe Systems – Guidance for Selecting the Appropriate Packaging Materials and for Siliconisation”. ONdrugDelivery, Issue 142 (Feb 2023), pp 66–74.
Bernd Zeiss addresses the influence of silicone oil on syringe systems and highlights the advantages and possibilities of novel silicone-oil-free prefillable syringes, both for glass and for plastic syringes.
The degree of siliconisation is just one of many aspects for consideration when selecting the right primary packaging material. Prefilled syringes (PFSs) are usually siliconised on the inside to allow the plunger stopper to glide.1 Vials can also be siliconised on the inside to improve emptying.
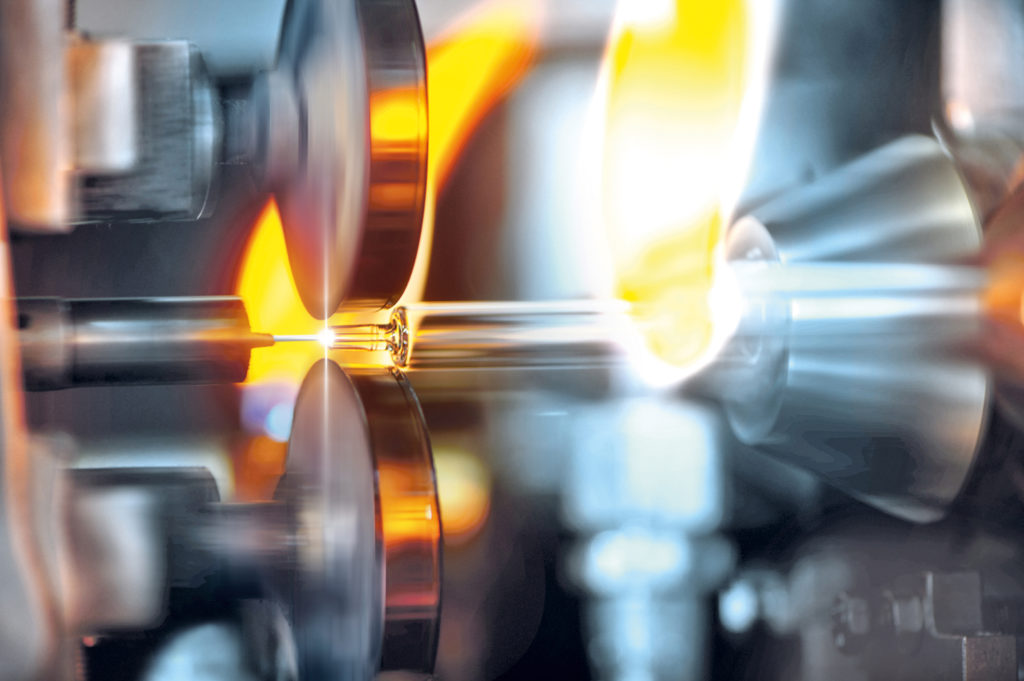
Figure 1: Tungsten pins are used as standard for defining the cone inner diameter of glass syringes.
VIAL OR SYRINGE
PFSs offer a number of advantages over traditional vials, which include considerably less effort required to prepare for injection and a reduced risk of application errors by specialist personnel or patients. The residual volume (dead volume) is also much lower for PFSs than for vials, even if disposable syringes – which have a low residual volume – are filled. Vials are always “overfilled” with a certain amount of drug to ensure complete withdrawal of the specified dose. One of the disadvantages of PFSs over vials, however, is that comparatively more materials come into contact with the liquid drug during storage. Besides glass and the elastomer stopper, these materials also include silicone oil as a lubricant for the stopper, usually another elastomer for the cap and possibly traces of tungsten, which can interact with the drug.2
Tungsten pins are used in glass syringe production to define the bore in the cone (Figure 1). Some advantages and disadvantages of PFSs and vials are listed in Table 1. Novel stoppers have also eliminated the need for silicone oil in glass syringes, which is particularly important for sensitive formulations and in ophthalmology (Figure 2).
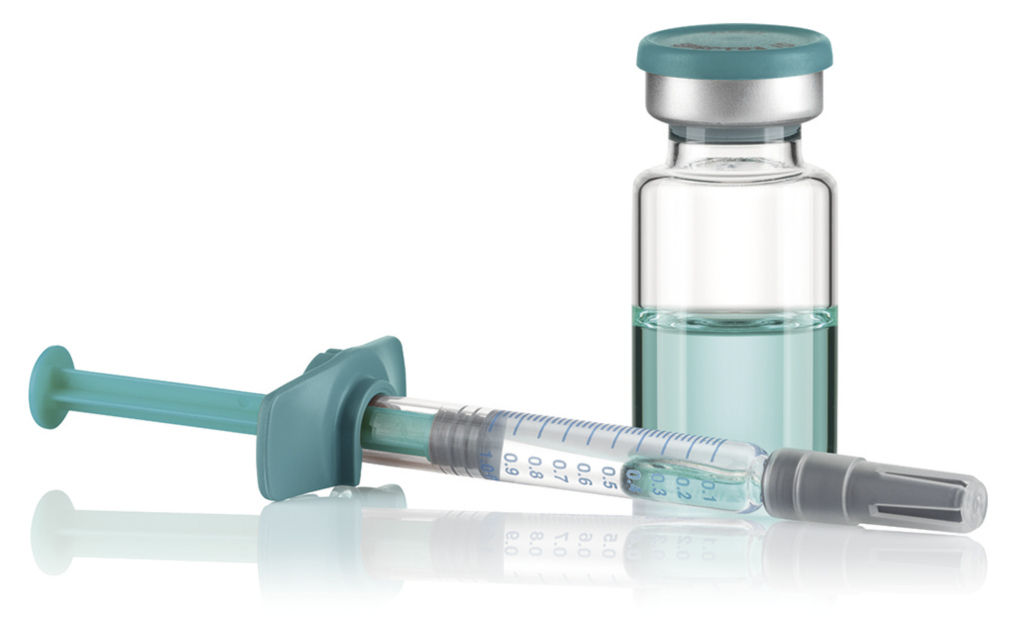
Figure 2: Vial, closed with rubber stopper and crimp cap. Syringe closed with needle shield and stopper, with plunger rod and “backstop”.
Prefilled Glass Syringe | Advantage | Filled Glass Vial, Closed | Advantage |
Total cost for container | |||
Low overfilling, low residual volume | + | High overfilling, high residual volume | – |
Higher costs for packaging materials | – | Lower costs for packaging materials | + |
User-friendliness | |||
Single dose | + | Single or multiple dose | ± |
Few steps through to injection | + | Many steps in injection preparation | – |
Low risk of incorrect dosing | + | Higher risk of error for correct dosing | – |
No other components needed (needle syringe) at point-of-care, except for: push-on cannulas for Luer syringes |
+ | Disposable components necessary at the point of care: Plastic single-use syringe Cannula for filling Injection cannula |
– |
Contact materials | |||
Contact with the drug during storage: Glass Elastomer stopper Elastomer cap Tungsten (extractables) Silicone oil (glide agent) Needle adhesive, Stainless steel |
– | Contact with the drug during storage: Glass Elastomer stopper |
+ |
Special applications | |||
High-viscosity drugs, low volumes | + | High-viscosity drugs | – |
Lyophilisation: reconstitution complex | – | Lyophilisation: reconstitution simple | + |
Autoinjectors are simple to use for at-home use | + | Training necessary, especially for the uninitiated | – |
OVERALL ADVANTAGE | 7+ 3– | 3+ 6– |
Table 1: Overview of some advantages of PFSs versus vials. The advantages for PFSs predominate. Case-by-case considerations are necessary for selecting the appropriate packaging material.
“The emergence of silicone-oil-free, adhesive-free and tungsten-free plastic syringes around 10 years ago was a challenge for glass syringe manufacturers, who had previously only competed among themselves.”
WEIGHING UP THE ADVANTAGES OF GLASS AND COP
Silicone-oil-free PFSs are not fundamentally new; they have long been available as cyclic olefin polymer (COP) plastic syringes from various suppliers.3 The advantages of plastic syringes are their resistance to breakage, absence of adhesive in the case of needle syringes and their very tight manufacturing tolerances. Disadvantages include the inferior barrier against gases and the specific plastic-extractables profiles as compared with conventional Type-1 glass. Table 2 provides an overview of the most important criteria.
Advantage of Glass |
Advantage of COP |
Remarks | |
Risk of breakage during filling | ± | ± | Line clearance after glass breakage during filling is expensive but rare |
Risk of breakage at the point of care | ± | ± | Possible, but rare with small volume syringes. Breaking force minimised in advance during development |
Luerlock integrated | – | + | Slipping of the thread and detachment impossible with COP |
Tungsten | – | + | Alternative pin materials available today, no tungsten in COP injection moulding |
Adhesive | – | + | COP syringe free of adhesive |
Silicone oil | ± | ± | COP syringes silicone oil free, long available |
Gas and especially oxygen barrier | + | – | Glass unsurpassed |
Extractables | + | – | Low for glass and known, inorganic |
pH shift | – | + | No pH shift with COP |
Experience | + | – | Experience with glass in the pharmaceutical industry is extensive, also for filling lines |
Costs | + | – | COP more expensive than glass |
Design freedom | – | + | Injection moulding allows diverse designs |
Tool | + | – | Free moulding needs no special, expensive injection moulding tools |
Tolerances | – | + | Glass with wider tolerances through free moulding |
Scratch resistance | + | – | Plastic sensitive, however scratches do not affect the breaking force |
Sterilisation of the packaging material | ± | ± | Glass: EtO COP: gamma, steam |
Terminal sterilisation | ± | ± | Glass: steam, EtO, other methods COP: steam, gamma, other methods |
OVERALL ADVANTAGE | 6+ 6– | 6+ 6– |
COP = Cyclic Olefin Polymer EtO = Ethylene Oxide
Table 2: Advantages of glass versus COP as primary packaging material for syringes and vials. Case-by-case considerations of the advantages of the syringe material must be given depending on the formulation and the field of application.
“The lower the number of materials in contact with the drug, the lower the risk of failure in stability studies.”
The emergence of silicone-oil-free, adhesive-free and tungsten-free plastic syringes around 10 years ago was a challenge for glass syringe manufacturers, who had previously only competed among themselves. This challenge was accepted and glass syringes have been significantly improved by many technical innovations as a result:
- Complex camera technology combined with special software allows extremely precise dimensional controls.
- Cosmetic defects that can cause glass breakage can be detected and minimised by special cameras and scanning algorithms.
- Design adjustments, such as small round finger flanges, further reduce the risk of breakage, e.g. for autoinjector applications.
Risks are minimised during product development, validation and quality control well before the market launch:
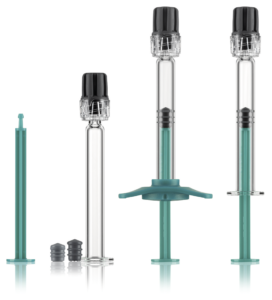
Figure 3: Ophthalmology 0.5 mL syringe with Luer lock adapter, BOS or silicone-oil-free, ready-to-fill format, various elastomer components and dose mark available. Gerresheimer syringe with TELC.
- Tungsten pins can be replaced by ceramics or other materials, which means that tungsten-free syringes are available today.
- Needle adhesives, with their narrow extractables profile, are now a reliably assessable risk for pharmaceutical companies. Special dry-needle systems can always be used as an alternative.
- Luer lock adapters on glass syringes have been optimised in terms of their twist-off and pull-off forces – see the Tamper Evident Luer Lock Closure (TELC) syringe (Figure 3).
- Silicone-oil-free prefillable glass syringes are now also an option. Special plunger stopper materials display good gliding properties and ensure container closure integrity (CCI) during storage, which is a key concern. Ultimately, the emergence of plastic syringes has improved glass syringes. Glass still has a market share well in excess of 90% compared with plastic syringes for small injection volumes primarily for intramuscular or subcutaneous use.
ADVANTAGES OF SILICONE-OIL-FREE SYRINGES
Glass has been well investigated as a material for injectables and is widely used in vials, PFSs, cartridges (mainly insulin) and ampoules.4 Additional siliconisation is often straightforward, both for the drug during storage and for the patient, who still takes a small amount of silicone oil onboard with each injection. Until recently, only plastic PFSs were available, but this is now changing with the advent of glass PFSs. Although silicone-oil-free syringes do not serve a mass market and classic glass syringe systems continue to be siliconised, there are still a number of interesting fields of application for silicone-oil-free glass syringes – primarily in ophthalmology and the biopharma sector. Table 3 compares the advantages and disadvantages of silicone-oil-free syringe systems.
Advantage for Silicone Oil-Free Syringes | Advantage for Siliconised Syringes | Remarks | |
Drug | |||
Particle load in accordance with USP / Ph. Eur. |
+ | – | Silicone droplets contribute significantly to the total particle load |
Interaction of silicone oil with the drug | + | – | Silicone oil can interact with drug constituents in a variety of ways |
Inertisation of the polar glass surface | – | + | pH shift, delamination, protein adsorption on glass hitherto unknown for siliconised syringes |
Silicone oil is also injected | + | – | Adverse reaction in the patient, if applicable |
Functionality | |||
Break-loose and gliding force | – | + | Lack of gliding layer increases forces, greater scattering |
Constant break-loose and gliding force after storage |
? | ? | Possibly advantages of silicone-oil-free systems in the autoinjector, lower aging effect assumed** |
Proven and familiar rubber stoppers | – | + | Possibly advantages over new types of materials in the approval process |
F&F process | – | + | Special moulding sets and process adaptation in F&F necessary |
Integrity of the CCI system | – | ± | Lack of siliconisation may increase risk of leakage |
Filling level meniscus | – | + | Concave meniscus in silicone-oil-free syringes – larger air bubble |
OVERALL ADVANTAGE | 3+ 6– | 5+ 3– |
F&F = Fill and Finish ** Further studies pending
Table 3: Some comparative advantages of siliconised and silicone-oil-free glass PFSs. Case-by-case considerations of the advantages of the siliconised or silicone-oil-free syringe system must be given depending on the formulation and the field of application.
Ophthalmology
In ophthalmology, silicone-oil-free means, above all, a significantly lower number of particles. Injection into the eye is subject to strict total permissible particle count requirements in accordance with USP <789> and Ph Eur 2.9.19. The most important applications for PFSs are cataract surgery and intravitreal injections.
Cataract surgery involves removing the clouded lens. To prevent the remaining outer lens epithelium of the lens capsule from collapsing during removal, the resulting cavity is briefly in shape with a hyaluronic acid-based fluid prior to insertion of the artificial lens. PFSs are generally used to achieve this.
Injections into the vitreous body – intravitreal injections – have to be performed repeatedly depending on the clinical picture, especially in cases of wet macular degeneration with vascular endothelial growth factor (VEGF) inhibitors. This may lead to the accumulation of particles in the vitreous body and, therefore, in the field of vision.
Baked-on silicone (BOS) for PFSs5 for such purposes is now state of the art to meet stringent regulatory requirements and, ultimately, minimise impairment of vision due to the accumulation of silicone droplets in the eye. Here, it is important that the maximum particle counts specified in USP/Ph. Eur. refer to the filled PFS, with the particles from the glass container, the stopper, the manufacturing process and the drug itself also contributing (Table 4). The fewer the particles coming from the container itself, the more likely it is that the USP or Ph. Eur. conditions are met.
USP <789> Test on Particle Count | Light Obscuration | Microscopic Method | |||
Diameter | Diameter | ||||
Size of particles | ≥10 μm | ≥25 μm | ≥10 μm | ≥25 μm | ≥50 μm |
Number allowed per mL | 50 | 5 | 50 | 5 | 5 |
Table 4: USP <789> and Ph. Eur. 2.9.19 prescribe maximum values for subvisible particles in ophthalmic applications. Depending on the measurement method, particles ≥50 μm can also be detected.
Biopharma: Antibodies and mRNA Stability in PFSs
The requirements are less clear in the biopharma sector. The advantages of silicone-oil-free or siliconised systems depend on the specific API and its formulation. Monoclonal antibodies can be sensitive to silicone oil in their formulations,6 so silicone-oil-free syringe systems may be beneficial in these cases.
Injections at intervals of a few weeks do not lead to a significant accumulation of silicone oil in the tissue; in addition, silicone oil is harmless to the patients themselves and is considered inert and non-allergenic. Only in the case of more frequent injections, such as with insulin, could a silicone-oil-free injection offer advantages in reducing silicone oil deposition under the skin, which may be cosmetically relevant.
If formulations are sensitive to silicone oil, silicone-oil-free syringe systems are recommended. Sensitive drugs may also be sensitive to oxygen, shock or shear forces during injection through the needle and leachables from the elastomers.
Despite some advantages, silicone-oil-free plastic syringes have failed to become widely accepted on the market, which could be due to the poorer oxygen barrier of COP compared with glass. Formulations are generally designed to be stored in syringes before being administered and must remain stable throughout. This is researched in extensive stability and spiking studies before a drug goes on the market.7,8 Suppliers of PFSs offer a wide range of glass and COP test samples, such as for pharmaceutical R&D purposes, and can help customers find the best PFS for their specific requirements.
In some cases, liquid formulations react to silicone oil9 and may therefore be less well suited for siliconised PFSs. This appears especially true for the new mRNA formulations that need to be combined with specific additives.10
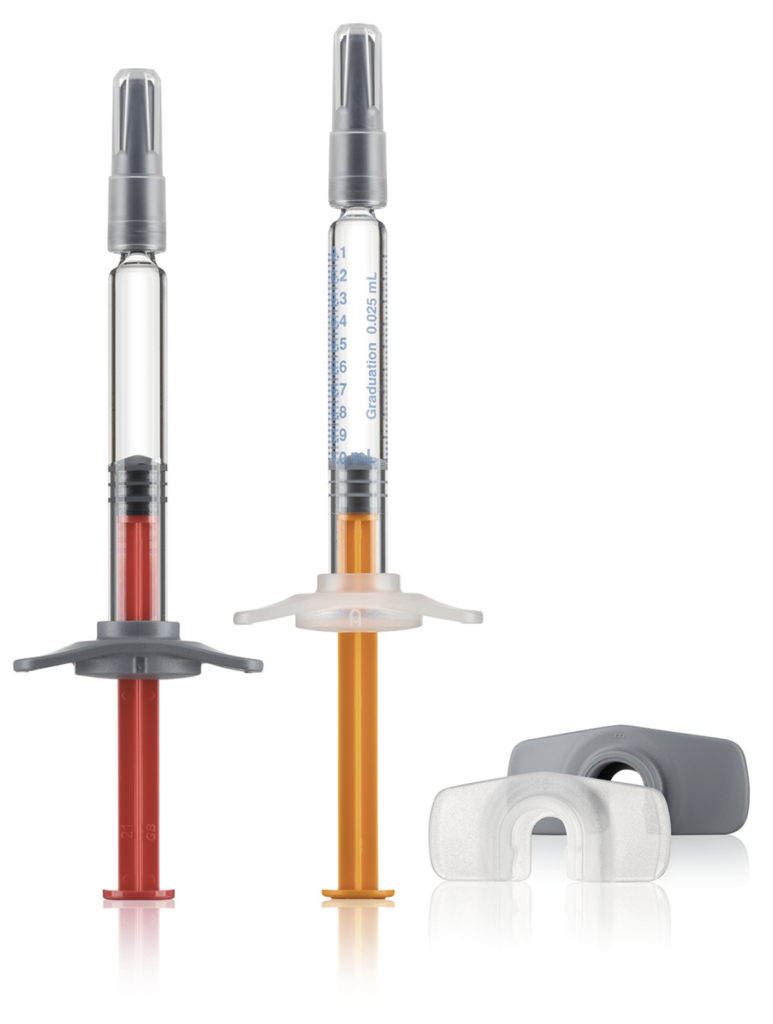
Figure 4: Gx Biopharma syringe Gx RTF 1 mL long needle syringe, low silicone level or silicone-oil-free, specified tungsten level, low specified extractables (elastomers), adaptation to autoinjectors, elastomer components possible.
The lipid nanoparticles (LNPs) used to introduce mRNA into cells could possibly be impaired by silicone oil. Besides LNPs, which are the vectors for introducing the mRNA into the cell, many other additives (excipients) are also needed in the formulation to stabilise the active substance. These are mainly pegylated or ionisable lipids, phospholipids, cholesterol, various buffers and salts.
Today, the new mRNA formulations are mostly stored frozen in silicone-oil-free vials for stabilisation prior to injection.
However, pharmacists aim to improve upon this by formulating drugs that can be stored as an unrefrigerated lyophilisate or, ideally, as a liquid formulation that remains stable at room temperature or under refrigeration (4–8°C) and thus could be made available in a PFS. This would significantly simplify handling, as with other classic vaccines, such as those administered in doctors’ offices.
Whether silicone oil, like oxygen, has a destabilising effect on mRNA drugs still needs further investigation. What is certain is that the lower the number of materials in contact with the drug, the lower the risk of failure in stability studies. Even though silicone oil is inert and does not directly impair the drug, the silicone layer applied as a lubricating coating can cause further problems.
Some liquid formulations dissolve the lubricating coating so that the break-loose and gliding forces deteriorate after some time.11 This is especially the case for autoinjectors, which empty the installed syringe with a specific spring force. This can lead to the undesired failure of the system after a certain period in storage. Pharmaceutical companies should not underestimate this risk, which will be investigated in the aforementioned stability studies prior to market approval. Silicone-oil-free syringe systems work without a coating that may be susceptible to change over time (Figure 4).
INVESTIGATIONS AT GERRESHEIMER
Gerresheimer has recently tested various silicone-oil-free syringe systems.12 Various systems have been initially evaluated in pre-studies. In addition to well-known providers whose products are already marketed,13,14 other providers were also considered. Extensive studies have demonstrated the suitability of the syringe systems that were investigated. The most important aspects of the investigations were particle load, CCI and functionality in terms of break-loose and gliding forces, including after storage. The stoppers should be freely available and also suitable for glass and COP syringes. The silicone-oil-free syringe systems on the market today are mainly available for COP syringes; in addition, the stopper and syringe body are only available from the providers as a fixed system. As a result of the diverse requirements for a syringe system, fixed combinations of stopper and syringe tend to be undesirable. The more flexibly a pharmacist can choose a stopper, the better they can respond to any difficulties in stability testing. The technical aspect of the fill-finish process should also be considered. Depending on the characteristics of the stopper, the setting tube method, vacuum setting or particular combinations of these may be considered. Without silicone oil, setting the stopper becomes even more complex.
Container Closure Integrity
Silicone oil performs two roles in a PFS – sealing and ensuring sliding while emptying. Integrity and integrity measurements represent a complex topic15 because different techniques can be deployed depending on the requirements – from testing in a dye bath (ISO 11040-4, Annex H) to sensitive helium leakage testing and beyond. All systems investigated met the basic requirements for PFSs – integrity testing in accordance with ISO 11040-4, Annex H was passed in all cases. Helium leakage tests were also carried out together with the stopper manufacturers, proving the integrity of the systems. Integrity tests with pharmaceutical formulations are pending, as they must be specifically drug-related.16
Particle Tests
The particle loads of the silicone oil-free syringe systems were determined in accordance with USP <788>/<789> and Ph. Eur. 2.9.19. Like many methods, the light obscuration particle count procedure is harmonised between the USP and Ph. Eur. USP <789> uses the same method, but with stricter particle limits, as shown in Table 4. For all silicone-oil-free syringe systems – glass or COP – and all stoppers investigated, the maximum particle values were significantly below the total permissible particles per container. The required values were also achieved for the established BOS syringes, and familiar, existing plunger stoppers can also be used. Further reduction in particle count is reaching its limits. Silicone-oil-free systems with specialised plunger stoppers have been developed to offer patients a PFS qualitatively improved in terms of particle load as well as the drug itself.
Figure 5 shows the particle measurement results for various siliconised and silicone-oil-free 1-mL-long syringe systems with limits in accordance with USP <789>. BOS syringes and non-siliconised syringes with novel stoppers (Plungers 1–3) are well within the non-critical range for intravitreal applications for all particle classes. Clear differences between the syringe body materials, (i.e. glass or COP) are not identifiable with silicone-oil-free systems. These syringes are suitable for ophthalmic applications.
Spray-siliconised syringes are generally not used for ophthalmic applications because the limits of 5 and 50 particles for the ≥10 μm and ≥25 μm size classes, respectively, are exceeded. A comparative measurement is included in Figure 5. Among the spray-siliconised syringes, it is noticeable that the COP syringes have significantly fewer particles in the ≥10 μm size class (52.5 versus 328.77 particles). The higher viscosity oil (12,500 cSt) used in the COP syringe bonds better to the glass and releases fewer particles that can be detected in the test liquid than the 1,000 cSt silicone oil classically used in glass syringes. This result may be of interest for non-ophthalmic applications.
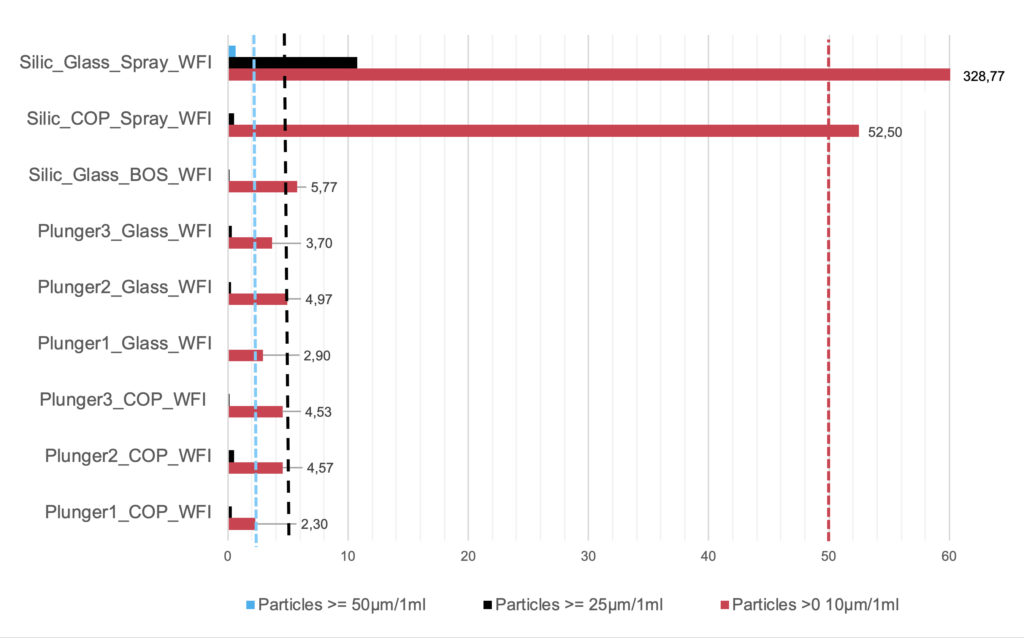
Figure 5: Particle measurements of silicone-oil-free 1 mL long syringes compared with siliconised (Silic) systems in accordance with USP <789>. Dashed = limits for three particle classes in accordance with USP <789>. Spray = spray siliconised, BOS; each with modern coated stoppers; COP/glass = syringe body material. Plungers 1–3 = silicone-oil-free syringe systems with various special stoppers. WFI = syringes filled with water for injection.
Break-Loose and Gliding Forces
Silicone-oil-free syringe systems face special challenges in the absence of a lubricant coating. Integrity must not be impaired but, at the same time, the break-loose and gliding forces must be ensured. Higher gliding forces can be generally expected without silicone oil than with classic siliconised syringes. Measurements at Gerresheimer were made with different stoppers in 1 mL long syringes three days after filling and stoppering, after three months of storage and after three and six months of accelerated ageing. Glass and COP syringes were investigated. Further data on 0.5 mL glass and COP syringes is also available or being acquired.
The results show good and fully acceptable break-loose and gliding forces for the silicone-oil-free syringe systems investigated (Figure 6). In particular, two systems showed very good results with hardly any changes during the storage period.
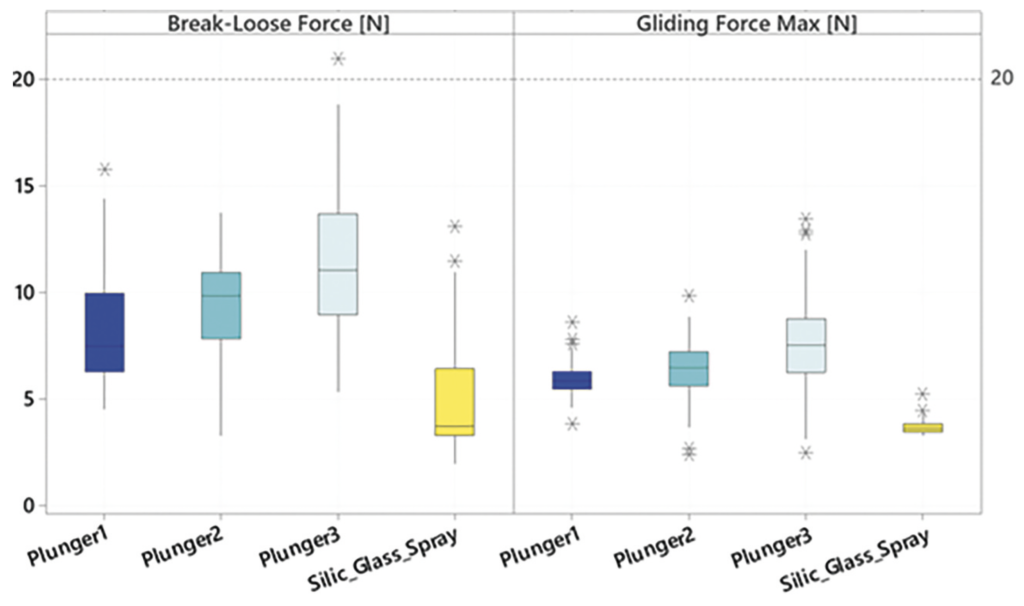
Figure 6: Break-loose and gliding forces of silicone-oil-free syringes compared with spray-siliconised syringes. Extrusion force 270 mm/min. Plungers 1–3: Three special stoppers from different manufacturers in unsiliconised syringes. Silic_Glass Spray: 0.5 mg silicone oil, coated stopper; 1 mL long 27 G needle syringe with standard ID, filled with WFI. Measurement times each with N = 160: summed [T0 (three days after filling), T1 three months, T1 acc (three months accelerated ageing in accordance with ICH), T2 acc (six months accel. ageing in accordance with ICH),
T2 (six months)].
Eliminating silicone oil expands the options for PFSs and will become increasingly important in the future. Besides ophthalmic and biotech applications, which primarily use 0.5 mL and 1 mL long syringes, 1, 2.25 and 3 mL syringes can also be used. The corresponding stoppers are available or are under development. Fill-and-finish (F&F) equipment manufacturers will add processability for silicone-oil-free syringe systems to their machines, and contract manufacturing organisations (CMOs) will gather experience in the filling process. Innovations in F&F will also generally require some time prior to implementation in the market because of the large number of interfaces that must mutually harmonise – from the successful development of a liquid drug, to the syringe manufacturer, to the stopper and machine manufacturer, through to the CMO.
REFERENCES
- Reuter B, Petersen C, “Syringe Siliconization, Trends, methods, analysis procedures”. TechnoPharm 2012, Vol 2(4), pp 223–244.
- Seidl A et al, “Tungsten-induced denaturation and aggregation of epoetin alfa during primary packaging as a cause of immunogenicity”. Pharm Res, 2012, Vol 29(6), pp 1454–1467.
- Dierick W, Yoshino K, “Using Prefillable Syringes for Biopharmaceuticals – Development & Challenges”. ONdrugDelivery, Issue 55 (Feb 2015), pp 10–16.
- Yoneda S, Torisu T, Uchiyama S: Development of syringes and vials for delivery of biologics: current challenges and innovative solutions Expert Opin Drug Deliv, 2021, Vol 18(4), pp 495–470.
- Wittland FD, Brandhorst E, “Method for producing prefillablesyringes”. US Patent Application US 2007/0186510 A1, 2007.
- Le Basle Y, et al, “Physicochemical Stability of Monoclonal Antibodies: A Review”. J Pharm Sci, 2020, Vol 109(1), pp 169–190.
- “PDA Technical Report No. 73 (TR 73): Prefilled Syringe User Requirements for Biotechnology Applications”. Parenteral Drug Association, Oct 2015.
- Guidance for Industry: “Immunogenicity Assessment for Therapeutic Protein Products”. US FDA, Aug 2014.
- Liu J et al, “Analysis of Silicone Oil in PFSs and Biopharmaceutical Drug Products Using High-Performance Liquid Chromatography”. AAPS PharmSciTech, 2021, Vol 22(2), p 75.
- Uddin MN, Roni MA, “Review: Challenges of Storage and Stability of mRNA-Based COVID-19 Vaccines”. Vaccines Basel, 2021 Vol 9(9), p 1033.
- Shi GH et al, “Impact of drug formulation variables on silicone oil structure and functionality of prefilled syringe system”. PDA J Pharm Sci Technol, 2018, Vol 72(1), pp 50–61.
- “Feasibility study silicone free syringe barrels. ”Gerresheimer: Internal Development report, 2021.
- “Silicone-free plungers to enable delivery of complex, sensitive biologics”. PDF, W L Gore & Associates, accessed July 2022.
- “Silicone oil and coating free stopper solution for prefillable syringes”. PDF, Injecto, accessed Jul 2022.
- Li L, “Container Closure Integrity Testing Method Development and Validation for Pre-filled Syringes”. PDA (2012): Universe of Pre-filled Syringes & Injection Devices Conference, Oct 16, Las Vegas, NV, accessed Jul 2022.
- Parenky AC et al, “Container Closure and Delivery Considerations for Intravitreal Drug Administration”. AAPS PharmSciTech. 2021, Vol 22 (3), p 100.