To Issue 132
Citation: Fraenkel E, Sørensen B, “Sustainability with the Aria Autoinjector: the Route to Further Improvements.” ONdrugDelivery, Issue 132 (Apr-May 2022), pp 46–53.
Emil Fraenkel and Bjarne Sørensen investigate the route to further sustainability improvements for the Aria autoinjector.
This article is a sequel to a benchmark study on the environmental impact of Aria presented by Phillips-Medisize in ONdrugDelivery Issue 126 (Oct/Nov 2021).
Though growing rapidly, the practice of developing and implementing circular products and business models is in an early stage. As a result, little research has been done on the application of circular design principles to specific industries and how the needs of those industries might affect circular design frameworks for their products. From an environmental perspective, studies have indicated that the healthcare sector accounts for 4.4% of global emissions.1 This needs to change, as has been clearly recognised by key stakeholders, including pharma and device companies as well as healthcare providers and patients. The business incentive for reducing emissions is less clear in some markets – Europe is starting to experience a real demand for more sustainable devices, while other markets are showing less concern.
This article considers the role of circular design in sustainable product development and its application to one such field: the medical device (MD) industry. In particular, it outlines the role that the remanufacturing of MDs can play in lowering the environmental impact of MDs. Using this principle and other aspects of circular design thinking, the article provides projections on how to further reduce the impact of the Aria autoinjector.
“Reusable autoinjectors, such as Phillips-Medisize’s Aria, consist of a disposable cassette, designed with recycling in mind, and a durable device, designed for longer use and suitable for refurbishment.”
APPROACHES TO IMPROVE PRODUCT SUSTAINABILITY
How can products be designed to be inherently good for both the future of the planet and the users that they are made for? This is a question increasingly being asked by product designers as more and more is understood about the environmental threats we face.
Traditional “design for sustainability” has attempted to minimise the pollution and carbon footprint caused by products. Today, we also recognise the importance of the raw material value that is lost and the environmental damage that occurs when products are manufactured from extracted materials, used and then disposed of in a single cycle.
In response to this, the idea of the circular economy2 has been developed, in which the reuse of products, or their component materials or subsystems, is considered. This is an idea which, starting from the 1970s, grew out of various schools of thought within economics, environmental science, engineering and design, and has been developed further by academic researchers and industry organisations ever since.
Despite all the efforts the health sector may make to decarbonise, some obstinate emissions will remain, even though they may get smaller over time. The industry must strive to ensure that these residual emissions are managed in a way that will support a healthier and more sustainable future.
The MD industry needs to be at the forefront of developing an approach to address these emissions by establishing bespoke health-based solutions that focus on reducing emissions at the source as a means of decarbonising.
Reduction of Emissions at the Source as an Alternative to Carbon Offsets
Lately, we have seen the development of carbon-neutral devices leveraging the sustainability megatrend and developed to achieve a zero-emissions position.3 The increased focus and awareness in the industry is positive but one should be careful with claiming absolute sustainability for devices as there is no such thing as an emission-free product. However, some products are less impactful than others and we should strive to minimise their environmental impact wherever possible.
Carbon-neutral products must rely fully or partially on compensating for their carbon footprint using CO2 certificates – often through third parties (e.g. reforestation programmes or investments in renewable energy) – a method that is heavily debated as to whether or not it has any significant positive impact on the environment. Typical offsets, like nature-based solutions (carbon sink enhancement), will not provide sufficient compensation for the level of residual management that is needed in the world and are often not considered sufficiently permanent or equitable.4
This is clearly a complex area of uncharted territory, with many ethical and practical pitfalls. The next step is for robust research into how such health-based solutions and interventions might support permanent emissions reductions that can meet the strictest criteria of standard offsets while avoiding their drawbacks.
Phillips-Medisize believes that designing for reduction, reuse and recycling will enable a more circular system where pharma companies, device developers and sub-suppliers must ultimately co-operate in taking back products for optimal repair, reuse, refurbishment and recycling (Figure 1).
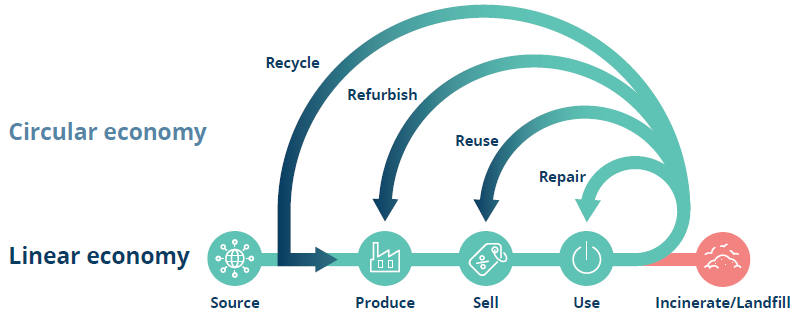
Figure 1: Linear and circular economy illustration – we should strive for tighter loops as repair is more advantageous than recycling.2
A transition to a circular economy is needed to reduce the overall impact of the industry. Reusable autoinjectors, such as Phillips-Medisize’s Aria, consist of a disposable cassette, designed with recycling in mind, and a durable device, designed for longer use and suitable for refurbishment.
OVERVIEW OF THE ARIA AUTOINJECTOR PLATFORM
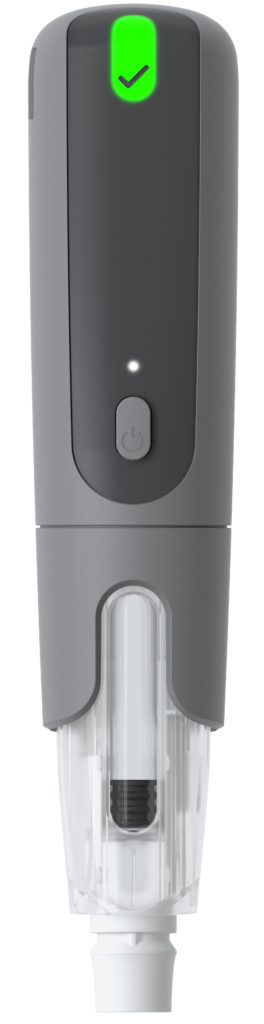
Figure 2: The Aria device and cassette.
Aria is a new smart autoinjector platform being developed by Phillips-Medisize to meet important emerging needs in the self-injection market, including improved device sustainability. The autoinjector is currently in development and not yet cleared by the US FDA and other medical device regulators. It consists of a reusable electronic power unit, which replaces the spring-powered drive in a mechanical device, coupled with a disposable cassette, which contains the prefilled syringe and provides needle safety, using a moveable shield, like most disposable devices. The cassette can accommodate both 1.00 mL and 2.25 mL prefilled syringes. There are two main models:
- Aria – which has a simple user interface
- Aria+ – which offers several advanced features, including a graphical user interface.
Both models include Bluetooth connectivity. Figure 2 shows the Aria device and cassette.
WE BELIEVE THERE IS POTENTIAL TO REDUCE THE CARBON FOOTPRINT OF THE ARIA AUTOINJECTOR BY 48%
In Phillips-Medisize’s previous ONdrugDelivery article on this topic,5 validated lifecycle assessment (LCA) data was presented, highlighting the Aria autoinjector’s potential CO2 footprint of 0.2 kg CO2 equivalent (eq) per injection. This figure was based on the scenario of a weekly injection over a device lifetime of three years, equivalent to a total of 156 injections.
The scope of the calculations and the assumptions here are the same as the calculations presented in the previous article and in Phillips-Medisize’s full LCA report, which is available upon request.
Phillips-Medisize’s projections show that refurbishment – a process in which devices are taken back, renovated, repackaged and then sent out for another lifecycle – will lower the environmental impact of the durable device. Changing the plastic material of the cassette to a bioplastic-based one will reduce the carbon footprint of the cassette significantly. Finally, incorporating the cassette into a recycling scheme will avoid emissions from new plastic being produced. Combined, these initiatives will potentially reduce the carbon footprint per injection by 48% (Figure 3).
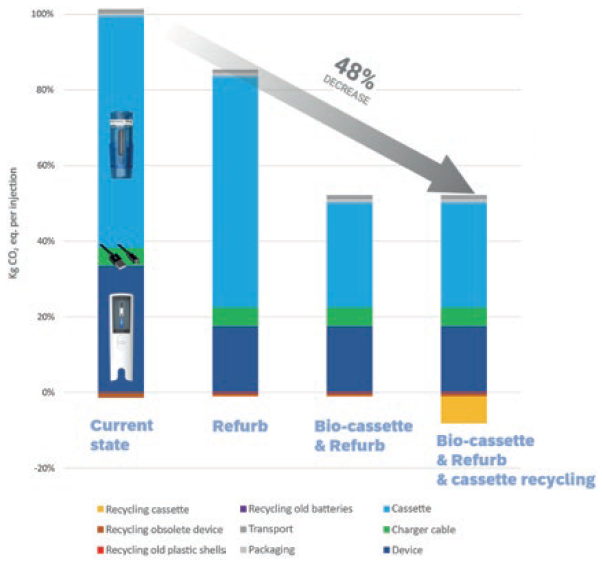
Figure 3: Overview of the impact of the current Aria autoinjector and the potential influence from different improvements.
REFURBISHMENT OF DEVICE
Manufacturers typically do not feel responsible for what happens with their products after customer use. Most products are designed in such a way that, while materials, assembly and distribution costs are minimised, their repair, reuse and disposal requirements are not considered. Manufacturers generally believe that the costs of incorporating these requirements would outweigh the benefits. Historically, most customers were not prepared to pay an additional fee for a “green” product6 – but the situation is changing.
Products that are obsolete or near obsolescence, such as through wear and tear, can be retrieved by manufacturers at the end of their lifecycle and put back into service by the replacement of crucial parts, a process known as refurbishing or remanufacturing.6 Figure 4 illustrates a process overview of what a refurbishment process could look like for the Aria autoinjector.
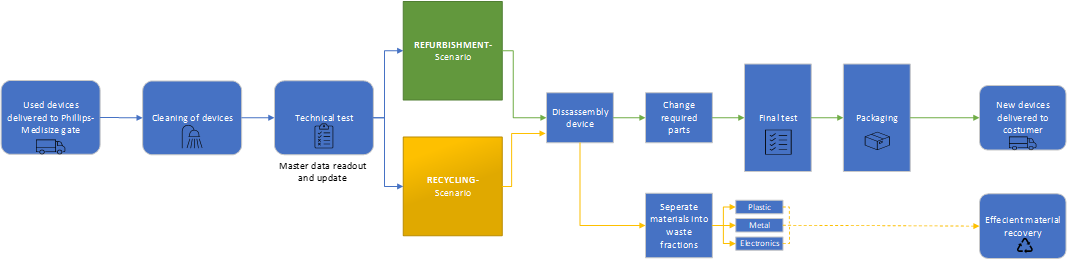
Figure 4: The refurbishment flow starts with devices being cleaned and tested. The device master data supplies information about the use cycle and can help determine if the device should be recycled due to high wear and tear or if there is a case for refurbishment, changing out worn parts like the plastic outer shell or the battery.
Phillips-Medisize can read out the device data containing key information about the actual use cycle and the number of injections the device has delivered, which is key for the refurbishment process. Based on the number of injections and state of the device, a decision procedure leads to either a “refurbishment scenario” or a “recycling scenario” determining the following process path:
“From an environmental perspective, products should be designed
for reuse as we ultimately want to keep products in the loop for as
long as possible.”
- Refurbishment scenario – a technical test, including data readout, will determine which parts need to be replaced. The outer casing of the reusable drive unit is likely to need replacement every time for visual appearance and hygiene reasons. The battery is another component likely to require replacement due to its limited lifespan. Any harvested components will be sorted into specific waste fractions for the best possible material recovery.
- Recycling scenario – even durable devices will reach a point where the producer cannot guarantee the function of the product due to wear and tear. However, the device still needs proper handling. Durable components, such as the electric motor, can be harvested for reuse in new autoinjectors or in other products. Hereafter, the device is dismantled and sorted into specific waste fractions for efficient recycling.
The final testing and packaging process is thought to be identical to the original process for a “virgin” device. Logistics scenarios and business aspects of the overall process will be determined in co-operation with the customer. With a refurbishment scheme as described, it is expected that the environmental impact of the Aria autoinjector will be reduced and that the device will sustain its useful life for a longer period of time.
In Phillips-Medisize’s own reduction projections regarding refurbishment of the Aria, the company bases the scenario on the device being refurbished once (exchanging shells and batteries), extending the lifetime from 156 injections to 312 injections, but further cycles of refurbishment are a possibility. The outer casing and battery are assumed to be recycled, and the obsolete device is assumed to be recycled by the consumer.
BIOPLASTIC CASSETTE
A substitution from a fossil-based plastic to bioplastic alternatives can be a relevant approach to lowering the carbon footprint of a product. However, while many things have been said about bioplastics, they certainly are no silver bullet to our plastic problem.
The first-generation bioplastic was based on feedstock from crops and plants, which is then removed from the food supply chain. Second-generation feedstock refers to crops and plants not suitable for human or animal consumption (food and feed). Second-generation feedstock can be either non-food crops (cellulosic feedstock) or waste materials from first-generation feedstock (e.g. waste vegetable oil).
Polyolefins can be made from bio-based feedstock, resulting in significantly lower greenhouse gas emissions compared with polyolefins made from fossil-based feedstock. The bio-based feedstock is often a waste material or residue from vegetable oils (second-generation bioplastics). The advantages of these bio-based polyolefins are that they have identical physical, mechanical and chemical properties, regardless of which feedstock was used in the production process – fossil- or renewable-based. This makes them suitable for medical appliances and can avoid expensive testing and documentation associated with new material selection.
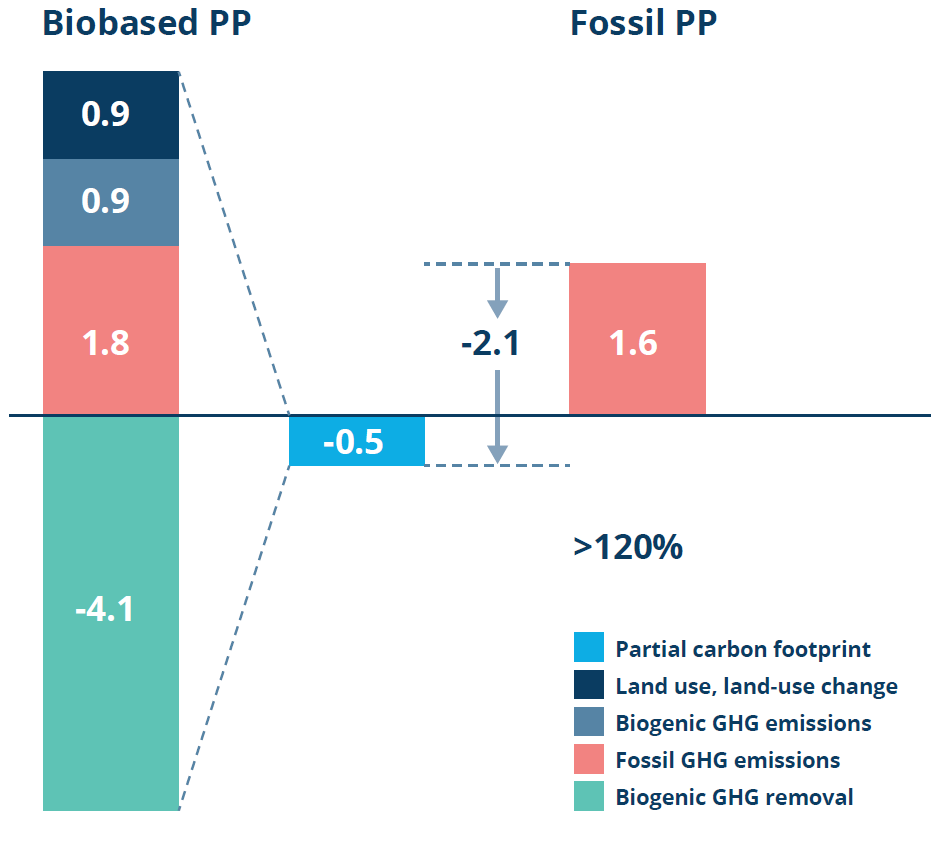
Figure 5: LCA data on the carbon footprint of biobased PP. (Data derived from a study carried out by the Institute for Energy and Environmental Research on Bornewables PP produced at Borealis’s sites using steam cracker or propane dehydrogenation – highlighting that the bio-based PP is a lower-emission alternative to fossil-based PP.7)
As an example of such a bio-based material, Figure 5 illustrates the lower footprint of bio-based polypropylene (PP) (-0.5 kg CO2 eq / kg PP) compared with traditional, fossil-based PP (1.6 kg CO2 eq / kg PP) – a decrease of >120% in carbon footprint. The reduced footprint is due to the stored carbon in a plant’s biomass, which it takes from the atmosphere during the plant’s growth phase. Calculations are based on the mass balance approach and is a cradle-to-gate consideration.
The emission data highlighted in Figure 5 serves as rationale for Phillips-Medisize’s reduction projections regarding bioplastic cassettes. Phillips-Medisize is currently investigating the possibility of supplying both bio and fossil-based PP cassettes.
CASSETTE RECYCLING
The sustainability of a product can be increased by designing for its end of life. In design for recycling, products are designed for easy disassembly and make use of recycling-compatible resins in material selection, allowing for easier material separation. Problems of plastic waste generation were taken into consideration at the design stage of the development of the Aria cassette. It is technically feasible to recycle the cassette, but there are some barriers that need to be addressed.
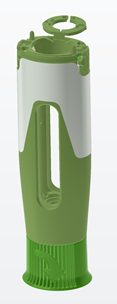
Figure 6: Aria cassette without syringe inserted, ready for recycling.
Barriers
From an environmental perspective, products should be designed for reuse as, ultimately, we want to keep products in the loop for as long as possible. However, in specific cases, other aspects can be of higher priority, such as safety in the case of a medical device. Potentially biohazardous waste products, such as autoinjectors, are likely to be classified as “hazardous waste”, which means that they must follow a strict disposal procedure, often being disposed of in sharps bins and then incinerated at special treatment plants.
The Aria cassette contains a prefilled syringe and needle, which is in contact with the patient and therefore assumed to be hazardous waste after use. From a regulatory point of view, there are restrictions on the transfer of this special type of waste, which can complicate recycling processes. The cassette (Figure 6) is needle safe, which could potentially allow it to come under a different classification of waste, thus allowing the possibility of recycling, either locally or as part of a take-back system that aims to reuse the material in new cassette production.
Carriers
The cassette is a mono-material design using PP for all components, reducing the number of different materials used from eight to just one (excepting the prefilled syringe), compared with typical disposable autoinjectors. While the syringe complicates the case for recycling disposable autoinjectors, the Aria cassette is designed with separation in mind. A locking mechanism keeps the syringe in place during use but can be unlocked in the disassembly process, allowing the syringe to be removed and the cassette body, a clean PP fraction, to be recycled into plastic granulate – preferably going back into the production of new cassettes to ensure circularity.
Assuming the cassettes are collected, an automated process for removing the syringe is perfectly feasible. The syringes can be recycled in a glass/plastic fraction. The syringe supplier, as an expert in its own product, could take back the syringe to ensure optimal recycling and potentially feed back the materials into its own production. Or, if this is not feasible with current designs, the material could be used for other applications.
Due to the versatility of PP, it is the most widely used type of plastic in disposable devices. This is an enabler for recycling – both in terms of recycling in local waste management systems and in potential take-back systems. If the material is not considered suitable for looping into the production of new cassettes, the PP fraction will still be a valuable fraction for products in other industries.
In Phillips-Medisize’s projections for improved sustainability through recycling, the company bases the scenario on the cassette being efficiently recycled into new granulate, thereby avoiding emissions associated with the production of new virgin granulate.
DESIGN STRATEGIES FOR IMPROVED MATERIAL CIRCULATION
Separation of recyclable waste and circularity of home healthcare MDs are new fields that are still being explored. The unexplored opportunities observed in this sector show the need for design strategies to expand the circularity of MDs. Recovery opportunities in the medical sector have been shown primarily to depend on the criticality of hygiene, product value and the environmental support structure, which affect infection control requirements on the one hand and the resources required for repair, refurbishment and recycling on the other. Figure 7 illustrates a matrix highlighting different design strategies, depending on product value/hygiene criticality.
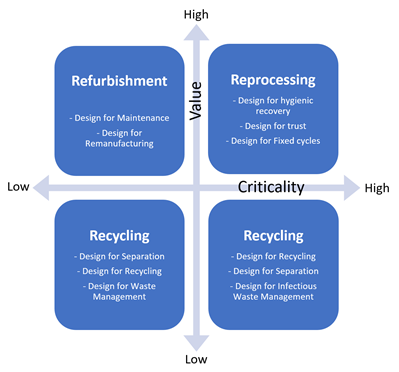
Figure 7: Design strategies for recovery of circular medical products in relation to product value and criticality – based on the work of Kane GM et al.8
Refurbishment of complex and expensive equipment, such as MRI and X-ray equipment, is already performed in practice,9 with well-documented design guidelines. In contrast, widespread hygienic recovery of high-criticality, medium-to-high-value devices is often performed poorly, with the design of such devices rarely accounting for such recovery and many of them unnecessarily being sold as “single use”.
Low-value, high-criticality products, such as disposable autoinjectors and the Aria cassette, are perhaps the most difficult subclass to develop a circular design strategy for, as they combine a high cost of recovery with a low product value and low cost of disposal and replacement. Design innovations for this group of products may be best targeted not at the products themselves but at the equipment and infrastructure required for their recovery. The Aria cassette is designed with recycling, separation and infectious waste management in mind. As with all high-criticality devices, extreme care must be taken when following this design strategy to ensure that patient safety is ensured.
High-value, non-critical products are those which can be reused without the necessity for hygienic recovery through aggressive sterilisation. These products are generally complex, durable pieces of equipment, such as Aria’s reusable electronic drive unit, and their designers can follow well-established principles for the design of long-life equipment and its refurbishment and maintenance. This means that products should be designed to facilitate the remanufacturing process, including component durability, design for disassembly and reassembly, accessibility, cleaning, reverse logistics and marketing.
Hatcher GD et al10 put together an overview of design concepts that are useful in design for remanufacturing: modularisation, platform design, active disassembly and failure mode. Design for refurbishment involves decisions related to the standardisation of parts, selection of durable materials and reversible fasteners.
CONCLUSION
By differentiating between the design strategy required for a reusable device and a disposable cassette, it is possible to optimise the sustainability aspects of the device and cassette even further to go beyond the benefits already attained though reuse of the device and minimisation of the materials used in the cassette. With a refurbishment scheme for the reusable device, it is possible to maximise the functional lifetime of the device’s constituent parts – and, when they reach the end of their lifecycles, the material recovery can be executed as efficiently as possible. From a commercial and sustainability perspective, this also means that the reusable approach is suitable for most injection frequency and stay-time scenarios.
From the outset, the Aria cassette’s size and weight have been minimised and further improvements to its sustainability profile are clearly possible, such as by manufacturing the cassette from bioplastic. Recycling scenarios for hazardous waste are more complicated, however it is technically easy to separate the syringe from the cassette in an automated process and harvest the glass and plastic material for reuse.
Generally, refurbishment and recycling are an area where global, as well as local, regulatory aspects can introduce challenges and obstacles that still need to be identified and further investigated. There are already options for servicing medical devices but, in the interest of broader sustainability improvements, regulatory hurdles could be challenged and potentially revised. Clearly, separating Aria’s drive unit from the disposable cassette, Phillips-Medisize has made it possible to pursue both optimum performance and connectivity options, while simultaneously minimising waste and carbon footprint per injection.
By making a full consideration of the principles of the circular economy, reusable devices like Aria can offer significant sustainability benefits compared with disposable devices.
REFERENCES
- “Health care’s climate footprint – how the health sector contributes to the global climate crisis and opportunities for action”. Green Paper, Health Care Without Harm, Sep 2019.
- “What is a circular economy?” Web Page, Ellen MacArthur Foundation, accessed Apr 2022.
- “World’s first zero carbon emission autoinjector from Ypsomed”. Press Release, Ypsomed, Oct 2, 2020.
- “Carbon offsets are not our get-out-of-jail free card”. UN environmental programme, Jun 2019.
- Fraenkel E, Sørensen B, “Sustainability with the Aria Autoinjector: a Lifecycle Assessment”. ONdrugDelivery, Issue 126 (Oct/Nov 2021), pp 36–44.
- Thierry M et al, “Strategic Issues in Product Recovery Management”. California Management Review, 1995, Vol 37(2), pp 114–136.
- “The Bornewables”. Company Web Page, Borealis, accessed Apr 2022.
- Kane GM et al,”Towards design strategies for circular medical products”. Resources, Conservation and Recycling, 2018, Article 135.
- “Behind the factory doors”. Company Web Page, Philips, accessed Feb 2017.
- Hatcher GD et al, “Design for remanufacture: a literature review and future research needs”. J Cleaner Production, 2011, Vol 19(17–18), pp 1909–2132.