To Issue 140
Citation: Deacon B, “Getting a Grip on Friction – a Crucial Consideration for Inhaler Design”. ONdrugDelivery, Issue 140 (Nov 2022), pp 64–68.
Bryan Deacon discusses the importance of considering friction when developing a new inhaler design, providing an overview of some of the basic considerations friction raises, as well as highlighting how optimising friction early with the aid of a materials expert can reduce risk, lower cost and lead to a better product overall.
WHY CONSIDER FRICTION?
There are always a wide variety of factors to consider for any design project, and inhalers are no exception. At the outset of a device design project, friction may seem like a safe factor to overlook as low priority and leave for later, to only deal with if and when it becomes a problem. However, to do so is to set the project up for expensive and time-consuming problems down the road when it becomes a fundamental flaw in the design, requiring late-stage redesigns and specialty materials to resolve.
“It is important to develop a solid understanding of how friction will affect a design as early in the development process as possible in order to avoid expensive mistakes down the line.”
Friction is not a trivial matter and is fundamental to the function and quality of an inhaler, be it ensuring the accuracy of a dose counter or guaranteeing that the complex mechanisms in a dry powder inhaler (DPI) work smoothly throughout their lifespan, from the first dose to the last. As such, it is important to develop a solid understanding of how friction will affect a design as early in the development process as possible in order to avoid expensive mistakes down the line. Partnering with a materials specialist like Celanese from the start can enable a simpler bill of materials, help avoid expensive mistakes, improve product quality and de-risk the whole development process.
Getting friction right isn’t just a question of making sure the mechanisms move as intended, but of ensuring that the end product is easy to use and of high quality. This is important for a multitude of reasons. For example, chronic obstructive pulmonary disease (COPD), one of the major indications treated by respiratory medication, primarily affects older patients, who may not have the strength to actuate their inhaler if the mechanism is too stiff or tends to exhibit stick-slip.
Friction affects not just the physical performance of a device but also its perceived quality. Sub-optimal friction pairings can make a device seem cheap and poorly made, potentially leading patients to lose confidence in its ability to deliver their treatment reliably and negatively impacting their compliance. It may even lead them to ask their healthcare provider for an alternative if they are especially dissatisfied.
Celanese can provide the expertise necessary to avoid the pitfalls of friction and ensure that a development programme progresses smoothly, using the right materials in the right way to achieve the functionality and quality required. This article covers some of the basic considerations of friction, providing insight into some of the questions that need to be answered during the development process to produce a device that stands out on the market.
KEY FRICTION INTERACTIONS IN INHALATION DEVICES
The friction interactions that will need to be optimised very much depend on what sort of device is being developed (Figure 1). The considerations for a single-dose DPI where the patient loads a capsule before each actuation are going to be different from those for a multidose DPI with a blister strip running over geared rollers, with the considerations for a pressurised metered dose inhaler (pMDI) being very different again. Knowing which mechanisms will be most impacted by friction is key to optimising the inhaler design.
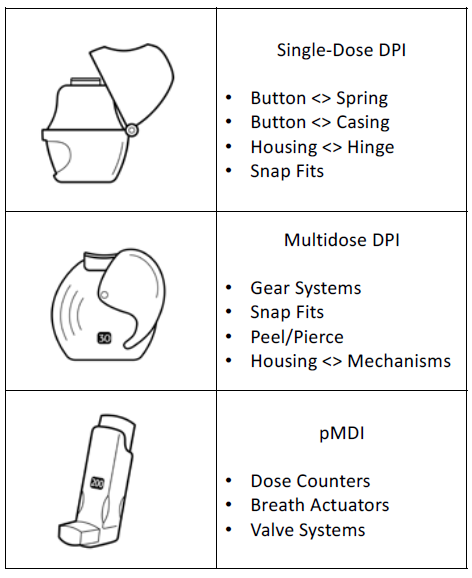
Figure 1: Key friction interactions for single-dose DPIs, multidose DPIs and pMDIs.
Single-Dose Capsule DPIs
Nearly all capsule-based DPI devices incorporate a manually operated spring-returned piercing mechanism. This piercing mechanism is critical for device function, so the design will need to be robust enough to guarantee that the mechanism works every time from the first to the last. Optimised friction in the mechanism can allow for the use of less stiff springs, an important factor in ensuring useability for those with impaired hand function. Lastly, the hinge should be considered as a point of quality – it neither wants to be too stiff, making it difficult to open, nor squeak, which can give the patient a poor impression of the device’s quality.
Multidose DPIs
Multidose DPIs are often based on complex gear trains to enable the peel and open/pierce mechanisms that move and open the blister strip. Failure in these mechanisms compromises the functionality of the device, be it by the mechanism jamming or slipping, leading to incorrect dose delivery. Understanding the friction at play in these gear mechanisms is therefore essential for ensuring that the device operates as intended. Friction must also be considered for dose counters, failure in which could, at worst, lead a patient to mistakenly believe that they have medication available when their device is empty, posing a significant risk to their health.
pMDIs
As with multidose DPIs, a key area of consideration for pMDIs is the dose counter. Ensuring that the dose counter is accurate is necessary for the device to achieve regulatory approval and guarantee patient safety. Additionally, advanced pMDIs may contain additional mechanisms where friction plays a critical role, breath-actuated pMDIs being a prime example. For a breath-actuated pMDI to function as intended, the friction in the release mechanism must be precisely calibrated so that the device activates with the force of the patient’s inhalation – too weak and it will activate at the incorrect time, too strong and it won’t activate at all.
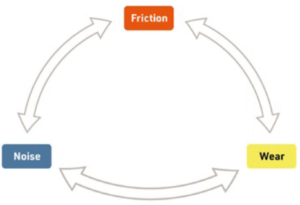
Figure 2: The friction-wear-noise triangle.
THE FRICTION-WEAR-NOISE TRIANGLE
Friction, noise and wear are all interlinked aspects of the operation of an inhaler, and understanding how they interact is key to understanding how a device will function over the long term (Figure 2). To begin with, friction, at the most basic level, comes from the interaction of intermolecular forces. The source of friction of primary interest in inhaler design is that which occurs between two surfaces as different parts of the device interact. Friction in this context considers how smoothly two surfaces move against each other and how much force is required to do so, as well as how those surfaces deform as they interact with each other.
Wear naturally occurs over time as a result of friction acting on the parts of a device, be it from fatigue, corrosion or fretting. For plastic contact, wear comes in two major types, abrasive and adhesive – scuffing and sticking. Abrasive wear reduces the friction between parts, which can impair functionality as parts cease to hold in place properly, slipping past each other when they shouldn’t. Conversely, adhesive wear means that parts stick together more strongly than they should, which can lead to jamming and increased stick-slip effects.
The third corner of the triangle is noise. There are two primary types of noise to consider here, squeaking and knocking. Squeaking is a result of the slip-stick effect. A completely smooth motion does not produce a squeak, whereas if the motion of one plastic surface over another is stop-start, with one surface sticking, then jumping forward, sticking again and repeating the process, it creates an audible squeak. Knocking, on the other hand, comes from mechanical interactions causing two parts to strike each other repeatedly.
Putting these three aspects together, noise can be viewed as an indication of unwanted friction within the device, which then results in unnecessary wear, which leads to more noise. Inhalers are rarely single-use devices, so controlling these three factors is key to producing a device that retains its functionality for its full lifespan and inspires confidence in its users – a patient might be unaware of the wear and friction happening inside a device, but they will certainly know if it starts making unwanted noise!
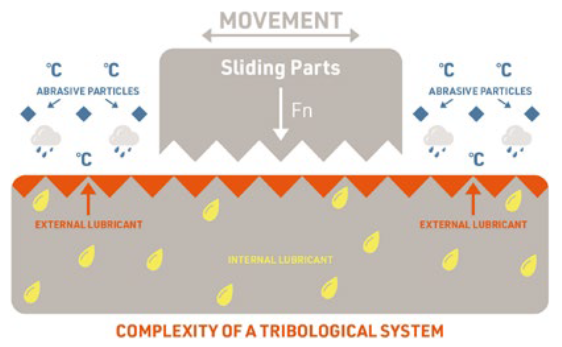
Figure 3: The total friction system.
UNDERSTANDING THE SYSTEM AS A WHOLE
To master the friction-wear-noise triangle, it is crucial to understand how friction acts upon a system as a whole (Figure 3). A fundamental aspect of this is a solid foundation in materials – knowledge of how different materials interact with each other and themselves is key to success. A good rule of thumb is to use dissimilar materials in combination for the best results, but the best and easiest approach is to engage with experts, such as Celanese, and make use of their existing knowledge base.
Even relatively simple systems will be dealing with multiple materials and a wide array of interactions. A brief list of key considerations for each of those reactions would include:
- Total sliding distance
- Contact force
- Sliding speed
- Type of interaction (e.g. continuous, oscillating, fretting)
- Surface finish
- Interaction environment (e.g. temperature, humidity, contaminants, etc).
“If friction considerations are ignored until a full prototype is developed, resolving friction-related issues can prove to be an expensive exercise, as part sizes may need to change or alternative specialist materials with the precise properties required need to be sourced.”
To characterise and understand each of these aspects, it is necessary to perform tests. If friction considerations are ignored until a full prototype is developed, resolving friction-related issues can prove to be an expensive exercise, as part sizes may need to change or alternative specialist materials with the precise properties required need to be sourced. If, instead, friction is considered from the start, testing can be performed using more standard materials to determine ahead of time which is the best fit for the device. To ensure the best results, either the same or the closest possible analogue material is used, which is facilitated by partnering early with a materials specialist.
Additionally, testing will be necessary to characterise breakaway forces and long-term wear. Breakaway friction plays a large part in how much force is needed to actuate the device, and so is especially important when designing a breath-actuated inhaler. Long-term testing characterising wear is necessary to ensure that the device will work as intended for its full lifespan. Both of these testing processes are easier and cheaper to complete if friction is considered early in the development process.
“Choosing the correct lubricant system for a given application requires understanding their various methods of action, strengths and limitations.”
LUBRICANT SYSTEMS
Even with careful selection of the most appropriate materials for an inhaler design, over the many times it will be used it is inevitable that some wear will occur. As such, it is important to consider how lubricant may help minimise the effect of that wear on device functionality. There are many lubricant systems available, including wax, chalk, silicone oils and polytetrafluoroethylene (PTFE), all with their own advantages and disadvantages (Figure 4). Choosing the correct lubricant system for a given application requires understanding their various methods of action, strengths and limitations. Here, making use of expert knowledge can be crucial for optimising device functionality, reducing risk and minimising cost.
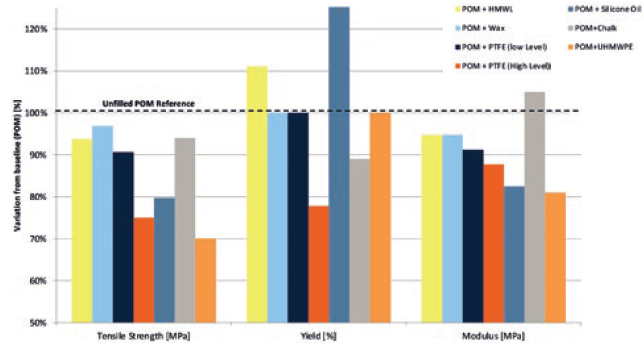
Figure 4: The effects on mechanical properties of various internal lubricants.
Immediate Versus Wear-Based Lubrication
The first big consideration when choosing a lubricant system is whether the lubrication needs to occur immediately from the first use of the device or be steadily introduced over time as the parts wear. Immediate lubrication provides its full benefit up front, but will diminish over time. This can be very useful if a little wear is needed to achieve the desired level of friction, while the initial use is too stiff. Wear-based lubricant, on the other hand, provides little to no immediate benefit, but instead is released as the parts wear. This means that lubricant is replenished at the point of interaction over time, providing a consistent force profile throughout the device’s lifespan.
“Working with an established expert in materials with a proven track record in tribology is the easiest and most effective way of capitalising on the advantages of optimising friction in your device.”
Internal Versus External Lubrication
Another significant dichotomy when it comes to lubrication systems is internal versus external. External is the simpler option, whereby a layer of oil or grease is applied to a surface at the point of friction during assembly, which can make the friction of the interaction largely independent of the materials. However, external lubricant is very susceptible to migration and can spread throughout the inhaler in undesirable ways, so thorough quality assurance testing is required to ensure that this is minimised.
In comparison, internal lubrication systems consist of small particles of lubricant contained within the material itself that are released as friction acts upon them. This means that internal lubricant systems are more easily controlled, but the cost of employing them can be greater than external systems. Which is more appropriate for a given application is therefore highly contextual. Developing a holistic understanding of how friction acts upon the system as a whole, along with the costs involved for both materials and testing, is absolutely necessary to optimise device design and assure regulators of its long-term functionality.
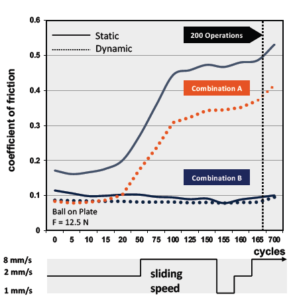
Figure 5: Development of friction over multiple movement cycles, as tested on an oscillating slip-stick tester.
CHANGES IN FRICTION OVER TIME
As has been discussed thus far, many factors affect the friction in any given material pairing. One of the most critical of these factors is the distanced travelled (or the number of cycles) an interaction will see – the balance between friction, wear and noise can vary significantly as sliding distance increases. As shown in Figures 5 and 6, while friction may stabilise during initial breakaway, it can change dramatically as the number of operations increases. Understanding the development of friction over time is specifically important in mechanisms such as dose counters and actuation triggers.
CONCLUSION – OPTIMISE EARLY
Whether it’s acknowledged or not, friction plays a major role in every inhaler development project. Understanding and optimising it early leads to reduced cost, lower risk and smoother regulatory approval. Working with an established expert in materials with a proven track record in tribology is the easiest and most effective way of capitalising on the advantages of optimising friction in your device.
As such an expert, Celanese can help ensure success. Using its broad portfolio of established medical grade materials, Celanese can help with materials testing, making sure tests are conducted with either the same material or the closest possible analogue to that intended for prototyping and production tooling. Furthermore, Celanese can assist in resolving problem interactions in the design, potentially offering modified polymer grades if required to achieve success.
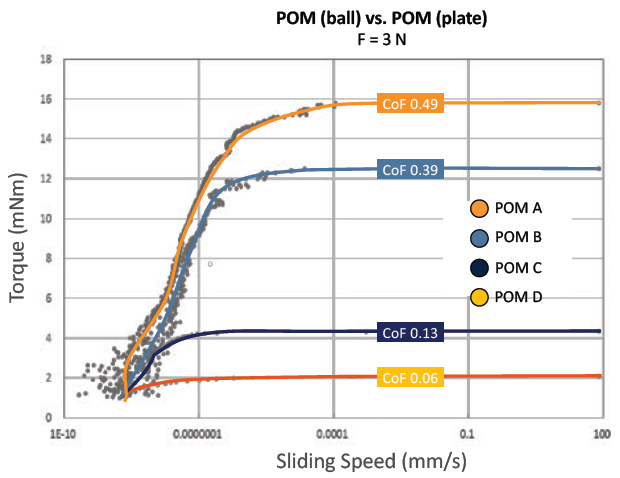
Figure 6: Initial breakaway friction and torque, as tested on a rotary MCR multi-tester.
Late-stage changes to inhaler design due to friction-related problems are costly to fix, often requiring multiple part changes, alternative materials or both. As such, partnering with an expert supplier early provides key benefits and expertise to the design process, helping generate designs that make the most of their materials and ensuring that the right questions are asked and answered before they become costly mistakes. Friction problems can easily sneak through the early stages of the development process, so getting a grip on friction reduces risk, lowers cost, improves quality and goes one step further to make sure an inhaler stands out against its competition.